John Lowengrub and colleagues provide insight into the challenge to eradicate glioblastoma and the use of stem cell transdifferentiation.
Huaming Yan1,7, Mónica Romero-López2, Lesly I. Benitez4, Kaijun Di4,9, Hermann B. Frieboes5,6, Christopher C. W. Hughes2,4,7,8, Daniela A. Bota8,9,10, Vittorio Cristini11, John Lowengrub1,2,7,8
1 Dept. of Mathematics, University of California, Irvine, CA 92697
2 Dept. of Biomedical Engineering, University of California, Irvine, CA 92697. Current address: Orthopedic Surgery, Stanford University, Redwood City, CA 94063
3 Dept. of Developmental and Cell Biology, University of California, Irvine, CA 92697
4 Department of Molecular Biology and Biochemistry, University of California, Irvine, CA 92697
5 Department of Bioengineering, University of Louisville, Louisville, KY 40292
6 James Graham Brown Cancer Center, University of Louisville, Louisville, KY 40292
7 Center for Complex Biological Systems, University of California, Irvine, CA 92697
8 Chao Comprehensive Cancer Center, University of California, Irvine, CA 92697
9 Department of Neurology, University of California, Irvine, CA 92697
10 Department of Neurological Surgery, University of California, Irvine, CA 92697
11 Center for Precision Biomedicine, Brown Foundation Institute of Molecular Medicine, University of Texas Health Science Center at Houston (UTHealth) McGovern Medical School, Houston, TX 77030, USA
Glioblastoma (World Health Organization Grade IV Astrocytoma, GBM) is the most aggressive and dangerous brain tumor. More than ten thousand GBM patients die each year in the United States, while the median patient survival for GBM is less than 12 months [1]. It remains a challenge to eradicate glioblastoma due to its high heterogeneity, intense vascularization and innate treatment resistance.
Over the past decades, various therapies have been studied and tested clinically. The standard of care treatment for newly-diagnosed GBM patients consists of surgical resection of the tumor, followed by combined radiation and chemotherapy [2]. Unfortunately, almost all the patients progress during or shortly after the treatment, and tumor recurrence is frequently observed. The FDA-approved antiangiogenic therapy for recurrent glioblastoma patients also fails to improve the survival rate [3]. This prompts a thorough understanding of the heterogeneity in glioblastoma, and the development of new, personalized and more effective cancer therapies.
In glioblastoma, the glioma stem cell (GSC) hierarchy has been found to play a crucial role in tumor development [4]. Tumors with high GSC population are more aggressive, and GSCs are believed to be responsible for tumor resistance to radiotherapy and chemotherapy [5]. Primary human endothelial cells (ECs) are found to help maintain the GSC pool and increase the size of tumor spheres, suggesting positive feedback from ECs to stem cells [6]. Furthermore, GSCs have been found to transdifferentiate into bona-fide vascular endothelial cells (GEC), which inherit mutations present in GSC, support GSC proliferation and self-renewal, and are resistant to traditional anti-angiogenic therapies [7].
In recent work, Yan et al. [8] have developed a 3-D hybrid continuum-discrete model of GBM that accounts for GBM cancer stem cells and their progeny, feedback among the different cell types, and tumor-induced neovascularization (Fig. 1A). Their model, which builds upon earlier work by Cristini and Lowengrub [9], shows that GSCs self-organize and GSC clusters emerge at tumor boundary (Fig. 1C). These GSC clusters generate invasive fingers with GSCs staying at finger tips and differentiated cells trailing behind, which has been observed clinically (Fig. 1B). Vessel sprouts form near the tumor boundary, then grow and anastomose into functional vessels that are connected to host vasculature and supply nutrients to the tumor. GECs form a network within the hypoxic core, consistent with experimental findings. In the tumor interior, the vessel density and the level of EC-GSC crosstalk is highest, resulting in multiple new GSC clusters. Consequently, cell proliferation is enhanced and the tumor volume grows rapidly. Since the EC-GSC crosstalk has fueled the tumor growth, it should be targeted in anti-tumor therapies.
Yan et al. first targeted the crosstalk using an extreme scenario in which the vasculature is removed completely from the tumor and the microenvironment, and new vessels are not allowed to form. When this anti-angiogenic therapy (AA) is applied continuously, the overall volume growth is reduced. The growth is driven by the GSC clusters at the finger tips, which are less affected by the removal of the vessels. Consequently, the fingers continue elongating and penetrating the host. Thus, AA considerably increases tumor invasiveness (Fig. 2, top), consistent with experimental and clinical findings [10].
Since GSC clusters near the tumor boundary plays an important role in tumor invasion driving invasive fingering, Yan et al. target these GSCs by modeling an anti-GSC therapy where the background vasculature continuously releases GSC differentiation promoters. Combining this differentiation therapy (Diff) with anti-angiogenic therapy, which has been shown to slow down tumor growth, also reduces invasiveness (Fig. 2, AA+Diff). However, the tumor cannot be eradicated since GECs are distributed at tumor boundary and protect GSC from differentiation (Fig. 2, yellow). When the tumor is treated additionally by an anti-mitotic therapy (e.g. chemo- and radiotherapy), the GECs still cover the tumor boundary and support GSCs (Fig. 2, AA+Diff+AM). Thus, Yan et al. turn to a combinatorial therapy that incorporates anti-GEC, anti-GSC (differentiation), anti-angiogenic, and anti-mitotic (e.g. chemotherapy) treatments. This combinatorial therapy is shown to be able to eradicate the tumor (Fig. 2, bottom), without recurrence even after the treatment is stopped, allowing the patient to enjoy true, long-lasting remission.
In these modeling studies, Yan et al. have shown that targeting the EC-GSC crosstalk may hold promise as a novel anticancer therapy. In addition, using combinatorial therapies to target transdifferentiated vascular endothelial cells present in glioblastomas could eradicate these deadly brain tumors without recurrence. One candidate agent is potentially an EGFR blocker. EGFR is involved in proliferation, differentiation, migration and angiogenesis regulation in many glioma tumors [11] and the EGFR mutation is also present in the GECs [12]. Therefore, blocking EGFR could effectively target GECs, among other targets. The latest generation of EGFR inhibitors, such as irreversible tyrosine kinase inhibitors (dacomitinib) effectively reduce the tumor volume in animal models by decreasing the GSC population by enhancing differentiation [13]. Therefore, Yan et al. suggest that a brain-penetrant EGFR inhibitor potentially matches the need for a differentiation promoter, anti-GSC and anti-GEC agent, which could be combined in future clinical trials with both anti-angiogenic therapy and chemotherapy.
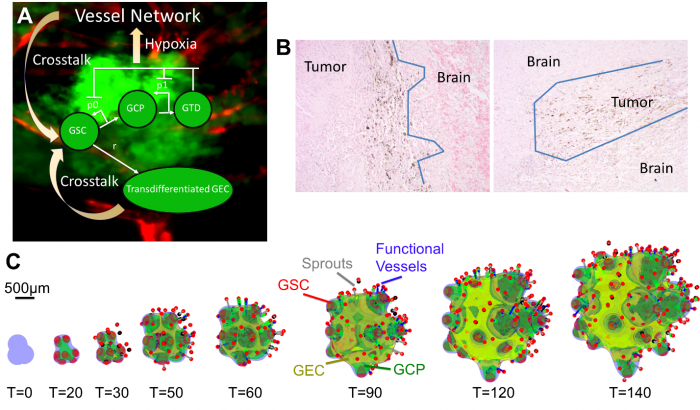
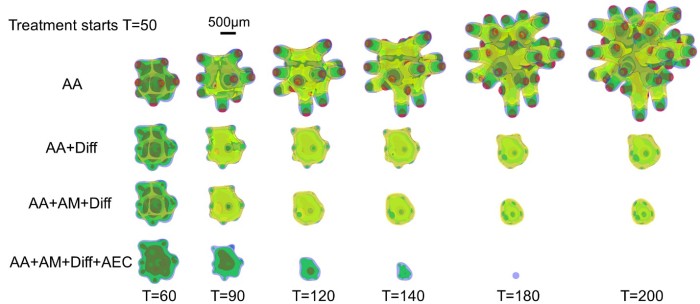
- Ostrom, Q.T., et al., CBTRUS Statistical Report: Primary Brain and Central Nervous System Tumors Diagnosed in the United States in 2008-2012. Neuro Oncol, 2015. 17 Suppl 4: p. iv1-iv62.
- Stupp, R., et al., Radiotherapy plus concomitant and adjuvant temozolomide for glioblastoma. N Engl J Med, 2005. 352(10): p. 987-96.
- Field, K.M., et al., Bevacizumab and glioblastoma: scientific review, newly reported updates, and ongoing controversies. Cancer, 2015. 121(7): p. 997-1007.
- Lathia, J.D., et al., Cancer stem cells in glioblastoma. Genes Dev, 2015. 29(12): p. 1203-17.
- Bao, S., et al., Stem cell-like glioma cells promote tumor angiogenesis through vascular endothelial growth factor. Cancer Res, 2006. 66(16): p. 7843-8.
- Calabrese, C., et al., A perivascular niche for brain tumor stem cells. Cancer Cell, 2007. 11(1): p. 69-82.
- Ricci-Vitiani, L., et al., Tumour vascularization via endothelial differentiation of glioblastoma stem-like cells. Nature, 2010. 468(7325): p. 824-8.
- Yan, H., et al., 3D Mathematical Modeling of Glioblastoma Suggests That Transdifferentiated Vascular Endothelial Cells Mediate Resistance to Current Standard-of-Care Therapy. Cancer Res, 2017. 77(15): p. 4171-4184.
- Cristini, V. and J.S. Lowengrub, Multiscale modeling of cancer: An integrated experimental and mathematical modeling approach. 2010, Cambridge, UK: Cambridge University Press.
- Paez-Ribes, M., et al., Antiangiogenic therapy elicits malignant progression of tumors to increased local invasion and distant metastasis. Cancer Cell, 2009. 15(3): p. 220-31.
- Roth, P. and M. Weller, Challenges to targeting epidermal growth factor receptor in glioblastoma: escape mechanisms and combinatorial treatment strategies. Neuro Oncol, 2014. 16 Suppl 8: p. viii14-9.
- Emlet, D.R., et al., Targeting a glioblastoma cancer stem-cell population defined by EGF receptor variant III. Cancer Res, 2014. 74(4): p. 1238-49.
- Zahonero, C., et al., Preclinical Test of Dacomitinib, an Irreversible EGFR Inhibitor, Confirms Its Effectiveness for Glioblastoma. Mol Cancer Ther, 2015. 14(7): p. 1548-58.
- Kaijun Di, M.E.L., Daniela A. Bota, TRIM11 is over-expressed in high-grade gliomas and promotes proliferation, invasion, migration and glial tumor growth. Oncogene, 2013. 32(42): p. 5038-47.
Contact information:
John Lowengrub
Chancellor’s Professor of Mathematics, Biomedical Engineering, Chemical Engineering & Materials Science
Director, Interdisciplinary Graduate Program in Mathematical, Computational and Systems Biology
540H Rowland Hall
University of California, Irvine
949-751-9700 (cell)
949-824-8456 (office)
949-824-7993 (fax)