Dr Alfred Msezane from the Department of Physics, Clark Atlanta University, tells us about the implications for electron affinity determination when considering metastable and excited negative ion formation in lanthanide and actinide atoms
A fundamental question we want to address and clarify here concerns the meaning of the electron affinities (EAs) for complex heavy systems, such as the fullerene molecules and the lanthanide and actinide atoms. The question is: Does the EA of these complex systems correspond to the binding energy (BE) of the electron to the ground, metastable or excited state of the formed negative ion during the electron collision with the target complex heavy system? Clarification of this issue should reduce the ambiguous and confusing meaning of the EA now permeating the literature. The meaning of the EA in the Au, Pt [1] and At [2] atoms as well as in the fullerenes C60 [3] and C20 through C92 is very clear. In all these systems the measured EAs have the same meaning as in the Regge-pole calculations. Namely, as the ground state anionic BEs of the formed anions during the collisions [4, 5].
For most of the lanthanide and actinide atoms, producing sufficient anions with known anionic BEs that can be used in photodetachment experiments is very challenging [6]. The lanthanide and Hf atoms provide clear cases of the ambiguous and confusing measured and/or calculated EA values [7, 8]. Fundamental to the reliable investigation and understanding of negative ion formation in complex heavy systems is the use of theoretical methods that account adequately for the essential physics, viz. electron-electron correlation effects and core-polarization interaction. These are fully embedded in our Regge-pole methodology.
-
Motivation
The paper has been motivated by:
- The need to understand and clarify the measured and/or calculated EAs of the lanthanide and the actinide atoms through low-energy electron elastic total cross sections (TCSs) calculations.
- The themed collection ‘Single Atoms as Active Catalysts’ celebrating the International Year of the Periodic Table, published by the Royal Society of Chemistry [9].
The fundamental mechanism underlying atomic negative-ion catalysis was proposed by our group in the context of muon catalysed nuclear fusion. The mechanism, requiring reliable EAs, involves anionic molecular complex formation in the transition state, with the atomic negative ion weakening the hydrogen bonding strength. The negative ion catalysis mechanism requires the delineation and identification of the ground, metastable and excited states anionic BEs of the formed negative ions during the collision of the electron with the complex heavy systems.
-
Lanthanide atoms
The lanthanide and the Hf atoms provide clear cases of the ambiguous and confusing measured and/or calculated EA values [7, 8]. As an example, for the Eu atom, two measurements determined different EA values for its EA, viz. 0.116eV and 1.05eV. These agree excellently with the Regge-pole calculated BEs for the highest anionic excited and the metastable states, respectively; the ground state anionic BE for Eu has been determined recently to be 2.63eV. Other examples where the measurements of the EAs correspond to the BEs of the metastable and/or excited states include the lanthanide atoms Tb, Tm, Gd, Nd and Lu as well as the Nb and Hf atoms [7, 8]. For Hf, the MCDF-RCI theoretical EA agrees excellently with the Regge-pole calculated BE of an excited state and not with that of the ground state. These results demonstrate the importance of obtaining definitive ground, metastable and excited ionic states BEs to avoid misidentification.
The recently reported conundrum in the measured EAs of the lanthanide atoms [7] stems out directly from the lack of unambiguous meaning of the measured EAs.
-
Actinide atoms
It is informative to discuss the EAs of the actinide atoms within the context of the most recent and first measurements of the EAs of both the radioactive Th and At atoms. The isotope 211At of the purely radioactive Astatine (Z= 85) is remarkably well suited for targeted radionuclide cancer therapy. The first measurement of its EA has been reported to be 2.416eV [2]. The EA of the unstable radioactive Th (Z=90) has been reported to be 0.608eV [10] as well. Indeed, these measurements particularly since both measured the EAs of radioactive atoms with close high Z values, obtaining significantly different EAs from each other, strengthen the debate about the meaning of the EA of complex heavy systems
In [11] the low-energy electron scattering from the actinide atoms Th, Pa, U, Np and Pu was investigated through the TCSs calculations. The objective was to delineate and identify the characteristic resonance structures as well as to understand and assess the reliability of the existing theoretical EAs (there were no measured EA values at that time). The recent measurement of the EA value of Th [10] warrants some discussion. The experiment [10] compared its EA with the calculated EA value of 0.368 eV [12], concluding that the measured value was about a factor of two larger than that calculated in [12]. However, in [11] we compared our calculated anionic BEs of the actinide atoms Th, Pa, U, Np and Pu with the calculated EAs, including those of [12]. For Th our anionic BEs are 0.549 eV and 0.905 eV and correspond to the highest and second highest excited states, respectively and not to the ground state, whose BE is 3.09 eV [11]. There is no reason whatsoever for the selective comparison by the experiment. In fact, our BEs above for the anionic excited states compare well with the calculated EA value of 1.17 eV [13].
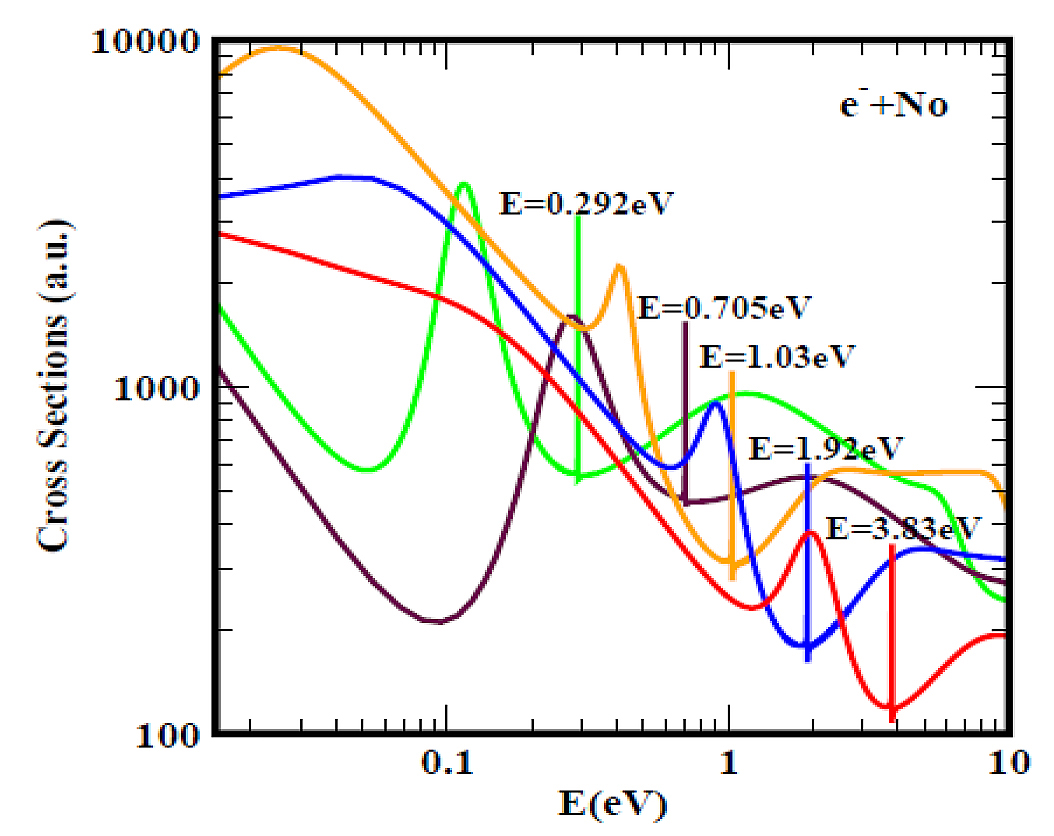
Figure 1 presents as an example, the typical electron elastic TCSs for the complicated No actinide atom. These TCSs, typical of those for the actinide atoms in [11], are characterised by ground, metastable and excited negative ion formation; they should be used to guide EA measurements. The red, blue and orange curves represent the TCSs for the ground and the two metastable states. The green and the brown curves are for the excited anionic states. Most important in Fig.1 is that the ground state BE of the formed negative ion during the collision corresponds to the EA of No atom.
Incidentally, many sophisticated theoretical methods struggle to obtain the ground state negative ion BEs of complex heavy systems such as the lanthanide and actinide atoms. Consequently, these theoretical methods tend to identify incorrectly the BEs of the metastable and/or the excited anionic states with the EAs. The recent measurement of the EA of the Th atom is such an example. Here, these ground state BEs are extracted from the Regge-pole calculated TCSs. For atomic Au, Pt and most recently At [2] – as well as the fullerenes C20 through C92 – the Regge-pole calculated ground state BEs matched excellently the measured EAs. Indeed, the methodology requires no assistance whatsoever from either experiment or other theory for the remarkable feat. These results give great credence to the power and ability of the Regge-pole method to extract reliable EAs from the calculated TCSs of complex heavy systems.
Concluding remarks
Many existing experimental measurements and sophisticated theoretical calculations have considered the anionic BEs of the stable metastable and/or excited negative ion formation to correspond to the EAs of the considered lanthanide and actinide atoms. The recent measurement of the EA of Th [10] is no exception. This is contrary to the usual meaning of the EAs found in the standard measurement of the EAs of such complex systems as atomic Au, Pt and At as well as of the fullerene molecules, from C20 through C92. In these systems, the EAs correspond to the ground state BEs of the formed anions during the collision.
In conclusion, there is remarkable agreement between the measured EA of At [2] and sophisticated theoretical EAs, see [14] for the extensive comparison. Indeed, our Regge-pole calculation demonstrated long ago that the EA of atomic At corresponds to the ground state anionic BE; similarly for other complex systems.
Acknowledgments
The research was supported by the U.S. DOE, Division of Chemical Sciences, Geosciences and Biosciences, Office of Basic Energy Sciences, Office of Energy Research. The computing facilities of the National Energy Research Scientific Computing Center are greatly appreciated.
References
- H. Hotop and W. C. Lineberger, J. Chem. Phys. 58, 2379 (2003).
- D. Leimbach, et al, arXiv: 2002.11418 (2020).
- D.-L. Huang, et al, J. Chem. Phys. 140, 224315 (2014).
- A.Z. Msezane and Z. Felfli, Chem. Phys. 503, 50 (2018).
- Z. Felfli and A.Z. Msezane, Euro Phys. J. D 72, 78 (2018).
- S.-B. Cheng and A.W. Castleman, Sci. Rep. 5, 12414 (2015).
- Z. Felfli and A. Z. Msezane, Journal of Atomic, Molecular, Condensate & Nano Physics 5, 73 (2018).
- A.Z. Msezane, Journal of Atomic, Molecular, Condensate & Nano Physics 5, 195 (2018).
- https://pubs.rsc.org › journals › articlecollectionlanding (2019).
- R. Tang, et al, Phys. Rev. Lett. 123, 203002 (2019).
- Z. Felfli and A. Z. Msezane, Appl. Phys. Research 11, 52 (2019).
- S.M. O’Malley and D.R. Beck, Phys. Rev. A 80, 032514 (2009).
- Y. Guo, and M.A. Whitehead, Phys. Rev. A 40, 28 (1989).
- R. Si and C. Froese Fischer, Phys. Rev. A 98, 052504 (2018).
Please note: This is a commercial profile