The electrical activity as a regulator of induction and the maintenance of specialised tissues is placed under the spotlight by Graciela A. Unguez at the Department of Biology, New Mexico State University in the United States
It is increasingly clear that electrical impulses – action potentials – have profound effects on virtually all organs regulated through neurons that communicate through such impulses. One such target organ that is regulated by nerve-dependent electrical impulses is the skeletal muscle invertebrate animals. In fact, the induction and maintenance of many properties of skeletal muscle are evident by the dramatic wasting and debilitating conditions that result in humans affected by genetic and degenerative diseases, trauma, injury and the ageing of the neuromuscular system1.
Conversely, we know that the application of neuromuscular electrical stimulation evokes involuntary muscle contractions and supports muscle mass maintenance in conditions of muscle disuse2. To date, research from many labs continues to uncover ways by which the phenotypic and functional properties of skeletal muscle respond to changes in innervation – under different environmental conditions from early development to ageing.
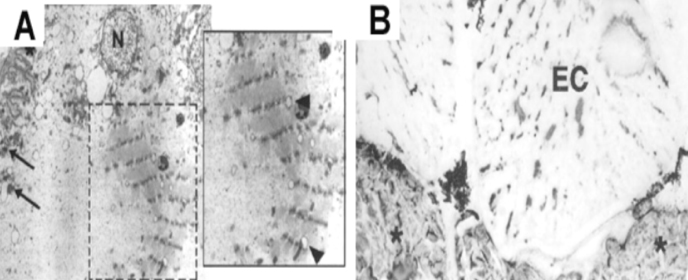
One fundamental question that remains largely unanswered is how do the amounts or patterns of electrical activation regulate the diversity of the many muscle fibre types? Specifically, how is neural input, coupled to the regulation of transcriptional and post-transcriptional changes of muscle genes that affect metabolism, morphology and contractility is unknown?
Moreover, the role that electrical activity patterns may plan in the extreme transformation of skeletal muscle from a force-generating tissue to a specialised energy-producing, but not force-generating, tissue has only recently been addressed. Here, an evolutionary myogenic “novelty” is highlighted to raise interest in phenotypic outcomes of distinct nerve-myogenic tissue systems maintained by unique electrical impulse patterns.
In the electric fish Sternopygus macrurus, some skeletal muscle fibres exhibit an extreme phenotypic plasticity by losing their contractility during normal development to give rise to electrocytes (ECs), the specialised cells that generate electricity and make up the electric organ (EO)3. ECs are also unique in that they are electrically driven by a population of spinal motoneurons called electromotoneurons (EMNs)4 at a continuous rate of 50-200 Hz5.
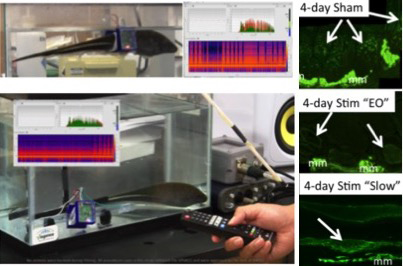
By comparison, somatomotoneurons (SMNs) in teleost fish innervate muscle fibres that are driven intermittently and at frequencies lower than 8 Hz6. We tested the idea that differences in activation patterns between SMNs and EMNs account for the differences in muscle and EO phenotypes in the adult7, by eliminating all motoneuronal activity to the muscle and EO tissues in adult fish.
Interestingly, this treatment resulted in the re-expression of sarcomeric contractile proteins and formation of sarcomeres de novo within mature electrocytes (Fig 1). Furthermore, in the absence of neural input the muscle-to-electrocyte conversion does not take place8.
To directly test the role of electrical activity on EC and its myogenic properties, we have pioneered a 3D-printed wearable backpack system that allows underwater chronic in vivo electrical stimulation (Fig 2)9. The stimulation pattern can be remotely controlled using infrared light with a TV remote controller. The stimulation pattern is adjustable by programming a micro-controller. The stimulator circuit is placed on a printed circuit board, which is placed in a waterproof circuit case attached to the backpack9.
Immunolabeling experiments of tails receiving different activity patterns suggest an upregulation of sarcomeric myosin heavy chain in ECs in sham controls (spinal transected only; Fig 2) and stimulated (spinal transected and stimulated; Fig 2) tails. The ability to impose a wide range of electrical activation patterns will further our knowledge of how specific features of electrical stimulation may affect differentiation, maintenance and trans-differentiation of the skeletal muscle program.
Other myogenically derived tissues with unique non-force producing functions such as the heater organ in billfishes10 and sound organ in toadfish11 also receive neural input, that is different from that of skeletal muscle fibres. Whether specific electrical impulse patterns induce and maintain the muscle-to-heater cell and muscle-to-sonic muscle cell phenotypes in these tissues will be interesting with respect not only the degree of plasticity of the skeletal muscle phenotype, but also the evolution of “novel” vertebrate tissues. Learning more about the electrical activity patterns in a wide range of nerve-target organ phenotypes is particularly relevant in the current interest in the development and use of electroceuticals – the use of electrical impulses to modulate the function and repair of body tissues12.
Funding source
This research supported by NIH grant 1SC1GM092297-01A1 and NSF INSPIRE Award CNS-1248109 (GAU).
References
- Robinson LR. (2000). Traumatic injury to peripheral nerves. Muscle & Nerve. 23:863-873.
- Dudley-Javoroski S and Shields RK. (2008). Muscle and bone plasticity after spinal cord injury: review of adaptations to disuse and to electrical muscle stimulation. J Rehabil Res Dev. 45:283-96.
- Unguez GA and Zakon HH. (1998a). Phenotypic conversion of distinct muscle fiber populations to electrocytes in a weakly electric fish. J. Comp. Neurol. 399:20-34.
- Bennett MVL. (1971). Electric Organs. In Hoar, WS. and Randall, DJ., eds., Fish Physiology, Vol. 5, Academic Press, pp. 347-491.
- Mills A, Zakon HH, Marchaterre MA and Bass AH. (1992). Electric organ morphology of Sternopygus macrurus, a wave-type weakly electric fish with a sexually dimorphic EOD. J. Neurobiol. 23:420-432
- Rome LC, Syme DA, Hollingworth S, Lindstedt SL and Baylor SM. (1996). The whistle and the rattle: the design of sound-producing muscles. Proc. Natl. Acad. Sci. USA 93:8095-8100.
- Unguez GA and Zakon HH. (1998b). Reexpression of myogenic proteins in mature electric organ after removal of neural input. J. Neurosci. 18:9924-9935.
- Unguez GA and Zakon HH. (2002). Skeletal muscle transformation into electric organ in S. macrurus depends on innervation. J Neurobiol. 53:391-402.
- Unguez GA, Duran C, Valles-Rosales D, Harris M, Salazar E, McDowell M, and Tang W. (2015). 3D-Printed Wearable Backpack Stimulator for Chronic in vivo Aquatic Stimulation. Conf Proc IEEE Eng Med Biol Soc. 2147-2150.
- Block BA. (1991): Evolutionary novelties: How fish have built a heater out of muscle. Amer. Zool., 31: 726-742.
- Nuki A and Somiya H. (2007): Innervation of sonic muscles in teleosts: occipital vs. spinal nerves. Brain, Behavior and Evolution, 69: 132-141.
- Famm K, Litt B, Tracey KJ, Boyden ES and Slaoui M. (2013). Drug discovery: A jump-start for electroceuticals. Nature, 496: 159-161.
Graciela A. Unguez, PhD
Professor of Biology
New Mexico State University
Tel: +1 (575) 646 7963
Please note: this is a commercial profile.