Dr Chunqiang Li, PhD from the Physics Department at University of Texas at El Paso explains the fascinating area of physics, optical microscopy in 3D
“Seeing is believing.” Of course, we must validate what we see as a full picture. Living in a 3D world, dynamic information obtained from 3D space will provide much more enriched information than that from individual 2D snapshots. Two-photon excitation has the advantages of reduced photobleaching, photodamage and background signal suppression compared with one-photon excitation and has been widely used in biomedical research, particularly for in vivo imaging. Laser scanning two-photon fluorescence microscopes are the workhorse. These commercially available microscopes typically can acquire two dimensional (2D) images in the range of one to one hundred images/second by raster scanning the laser beam in a sequential manner. Typically, the laser beam scanning is the bottleneck of acquitting high-speed imaging.
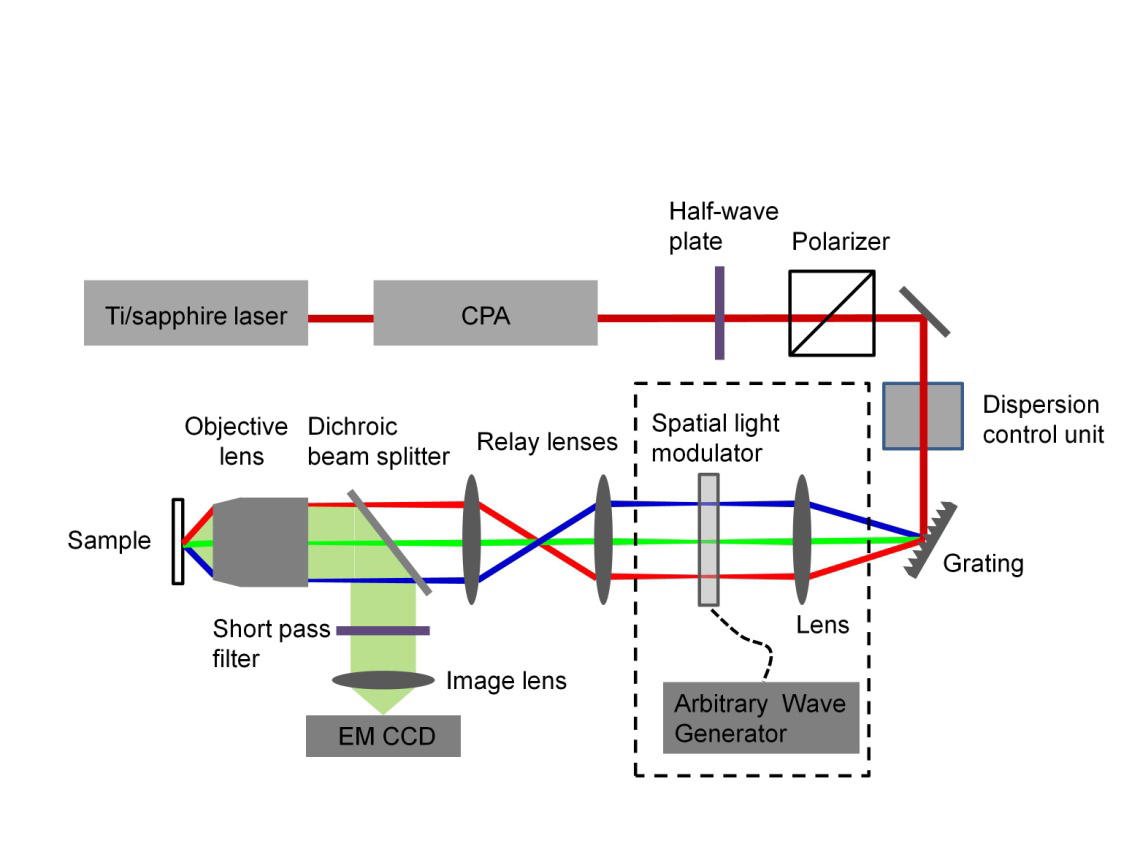
In the last decade, several groups have demonstrated temporal focusing as a way of implementing two-photon microscopy for wide-field 2D imaging, without scanning the laser beam. In a temporal focusing two-photon microscope, the focal region is a light sheet with a diameter around a few hundred micrometres, depending on the focal length of lenses and femtosecond laser pulses. which only reach its shortest temporal profile at focal region. Therefore, when using a CCD camera as a 2D detector, this temporal focusing two-photon microscope can obtain wide-field 2D images without scanning laser beams. Based on the success of this technique, several groups have adopted temporal focusing to image cellular dynamics in thick tissues. Since it does not require scanning the laser beam, it could reach 1000 frames/second 2D imaging speed with an amplified femtosecond laser system as the light source.
In many applications, especially in live animal imaging, it is necessary to acquire information in all three dimensions. To achieve 3D volumetric imaging, most modalities still need to mechanically move the sample stage or the objective lens to acquire depth information (z-scanning). In the temporal focusing two-photon microscope, it has been demonstrated that changing the group velocity dispersion (GVD) of the femtosecond laser pulses could lead to the displacement of the plane of the temporal focus, along the optical axis of the objective lens, yielding z-scanning as a function of GVD. Femtosecond laser pulse shaping is a technique that spreads femtosecond laser pulse spectrum in space, then modulates each wavelength component with a spatial light modulator (SLM) to arbitrarily shape an ultrafast pulse. Hence, pulse shaping could electrically control the GVD of the femtosecond laser pulse without any mechanical motion, which could further control the z-scanning in the temporal focusing two-photon microscope. Therefore, the goal of our project is to integrate pulse shaping into temporal focusing two-photon microscopy to achieve 3D volumetric imaging without mechanical scanning.
Figure 1 illustrates a schematic setup of the temporal focusing two-photon microscope. To achieve higher imaging speeds, a high peak power laser is needed. Therefore, a chirped-pulse amplifier (CPA) is added after the Ti:Sapphire laser to achieve high peak power (>1 uJ/pulse) at a repetition rate of more than 1 kHz. The incident angle of the grating is adjusted to ensure the central frequency follows the optical axis and propagates through the 4f setup, which comprises the collimating lens, relay lenses and the objective lens. Since the spectrum of femtosecond laser pulses has been spread out spatially by the grating, the next step is to add a pulse shaper (dash box in Figure 1) to use acoustic waves to control the dispersion of the femtosecond laser pulses in the spectral domain to achieve scan-less 3D volumetric imaging. The spatial light modulator (an acousto-opto modulator, AOM) is located at the 1f location to the left of the first collimating lens. The AOM is driven by a radio frequency arbitrary wave generator to create the desired radio frequency wave that controls the GVD of the femtosecond laser pulse.
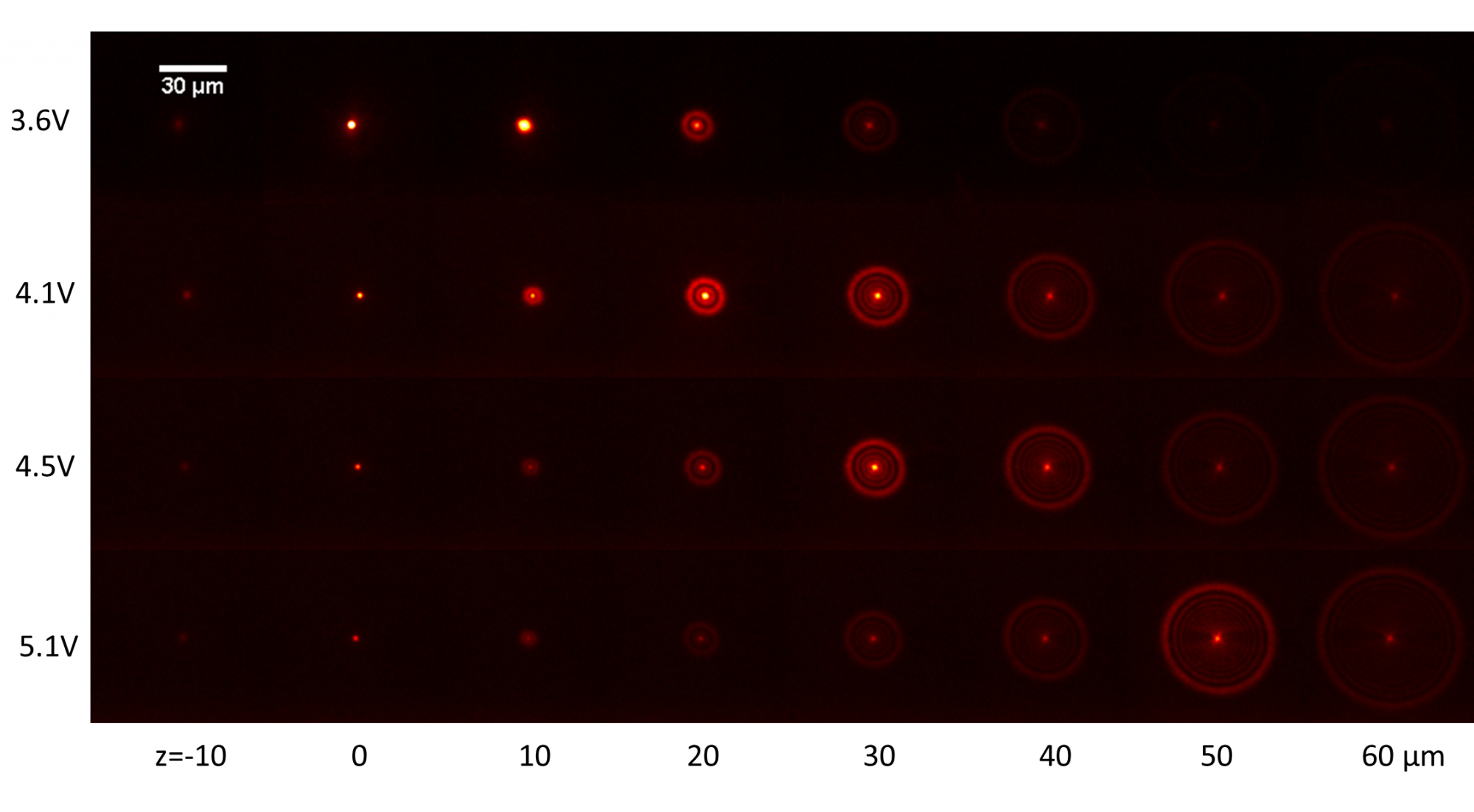
Our microscope demonstrated the capability of shifting the temporal focal plane via pulse shaping. We applied different voltages on the arbitrary function generator and the z position of the sample was scanned by moving the 3D sample stage with x and y positions fixed and finally, a fluorescence image was acquired at each z position. This varying voltage function induced GVD on the laser spectrum and changed the temporal focal plane, as shown in Figure 2. This shifting of the temporal focal plane by applied GVD depends on several parameters, such as laser spectral width and the numerical aperture of the objective lens. The temporal focal plane was shifted to z= 60 μm plane by applying a voltage of 10.2 V. This separation of the temporal focal plane from the geometric focal plane has a negative effect on the image quality. When the temporal focal plane is slightly shifted away from a geometric focal plane in the range of ±10 μm, the single fluorophore image becomes blurry. When this shift is larger, the single fluorophore image has the characteristics of concentric rings with peak intensity in the outermost ring and the central lobe becomes blurred as shown in the second to fourth row in Fig. 2.
Overall, we integrated ultrafast laser pulse shaping with the temporal focusing two-photon microscopy and achieved a high-speed 3D imaging method. This 3D imaging system requires neither laser beam steering nor sample mechanical scanning. The depth scanning is achieved by controlling the femtosecond laser spectrum. The dependence of scanning depth on the applied electronic signals which can be tuned at a millisecond timescale. Its high-speed 3D imaging capability was demonstrated by imaging fluorescence microspheres in a volume of 100×100×80 μm3.
Please note: this is a commercial profile
Dr Chunqiang Li, PhD
Physics Department
University of Texas at El Paso
Tel: +1 915 747 7537