Scientific progress to grow new tissues outside of the human body led to first market approvals of living tissue-engineered implants
The creation of living tissues to replace or repair damaged tissues or organs in the human body has defied clinicians and researchers for centuries. Yet, only by the end of the last century, scientific progress and systematic approaches to grow or culture new tissues outside the human body led to the first market approvals of living tissue-engineered implants.
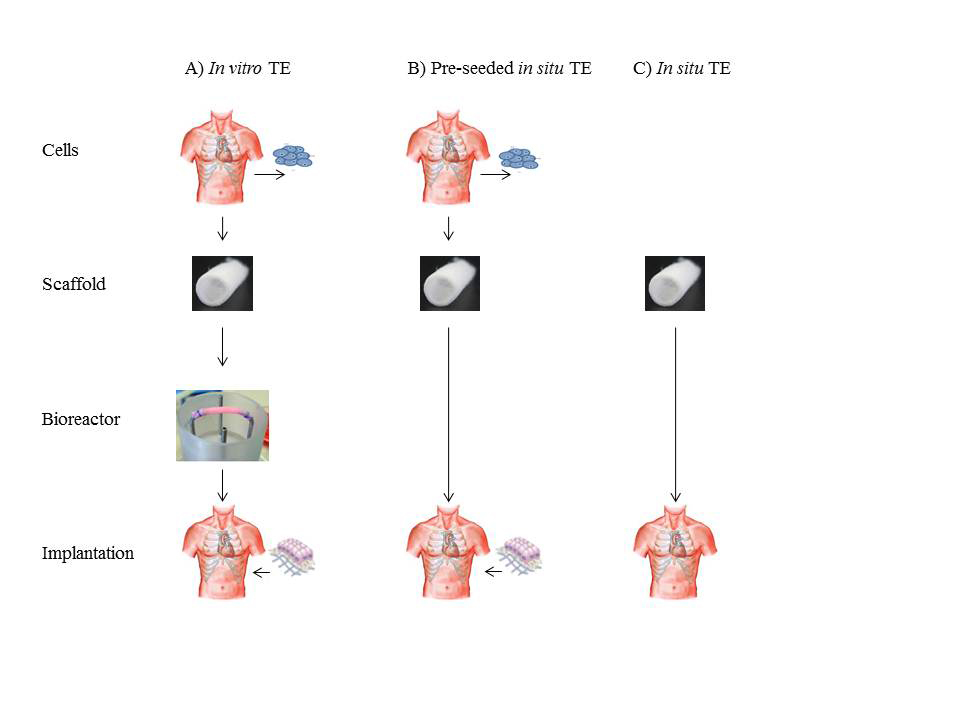
In its most fundamental paradigm tissue engineering entails the seeding of living cells, harvested from a donor, onto a pre-shaped biodegradable support material, or scaffold, of synthetic or natural origin. This cell-scaffold construct is generally cultured in a so-called bioreactor under conditions that favour cell expansion, tissue growth and tissue function. When the tissue has reached targeted functional properties, it can be implanted (Fig. 1A). Key to the success of this approach is the synthesis of substantial new tissue by the cells to take over the function of the degrading scaffold.
Compared to other approaches for tissue regeneration, like stem cell therapies, in vitro tissue engineering emerged as a promising therapy to replace tissues with a predominantly mechanical function that should withstand high dynamic loads immediately upon implantation. As such, research in our group concentrated on the creation of structurally organized load-bearing tissues for the cardiovascular system: functional blood vessels, load-bearing heart valves, and supportive cardiac muscle tissues.
Heart valves
A prominent example is the heart valve. Heart valves control unidirectional flow through the heart and their damage or malfunction often leads to serious medical conditions. End-stage heart valve disease is commonly treated by replacement of the valve with a mechanical or bioprosthetic device. While these prostheses generally improve survival and quality of life, they have technical drawbacks that limit their long-term efficacy. These include thrombo-embolic complications requiring lifelong anticoagulation therapy in case of mechanical valves, and limited durability due to calcification and structural failure in case of bioprostheses. Most importantly, prosthetic valves, including donor valves, are non-living structures that do not grow, repair or adapt. As a consequence, the life expectancy of patients after heart valve replacement is substantially lower than that of age-matched healthy individuals. The creation of a living, tissue engineered heart valve could circumvent many prostheses-related complications and would be particularly beneficial to paediatric patients.
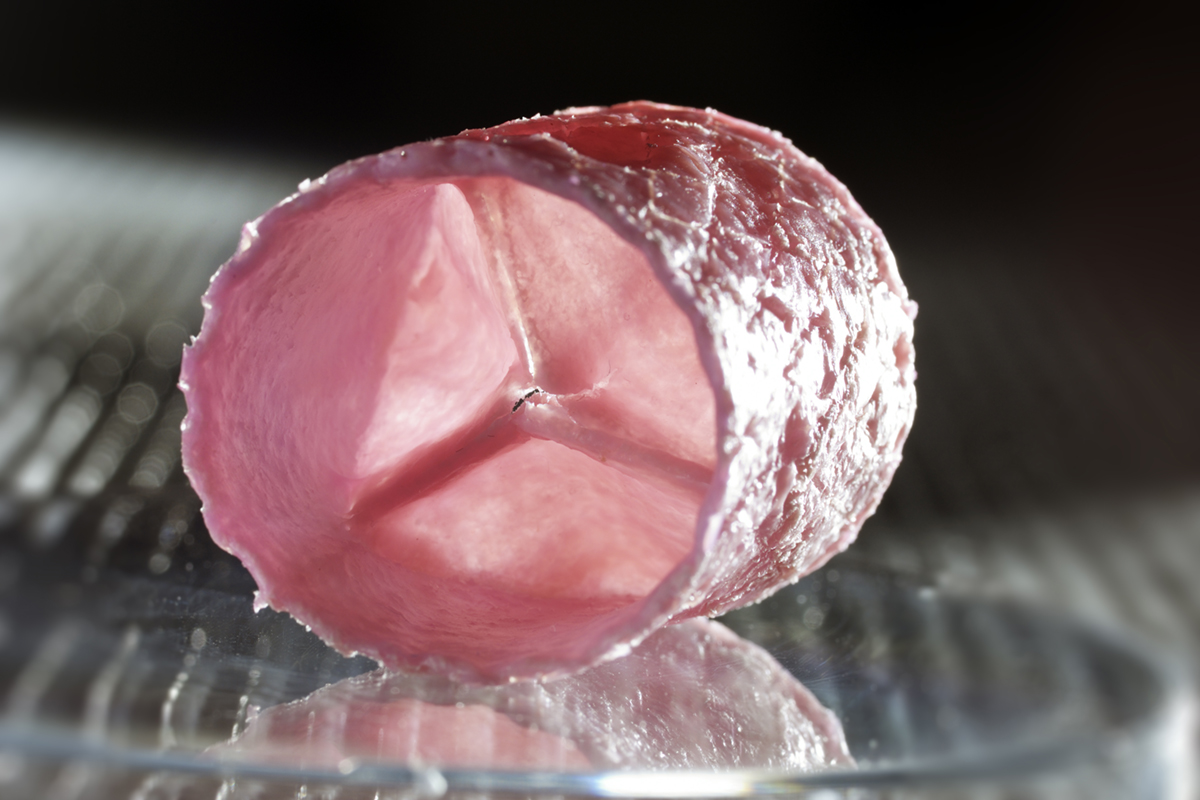
IMAGE CREDIT for Fig. 2 – Image: © Bart van Overbeeke
By merging insights from engineering, life sciences and medicine, our group, in collaboration with partners from the University of Zurich, was the first to develop a living tissue engineered heart valve that could withstand systemic loading and hence could function as an aortic heart valve1 (Fig. 2). Translation of this technology to the clinic, however, was hampered by suboptimal long-term in-vivo performance of these valves and valves from other labs – the biggest issue being valve leaflet retraction and consequent valvular leakage due to traction of the used cells. In addition, clinical introduction is hindered by the costly and laborious cell and tissue culture, associated high risks of infection, and the complex logistics and regulation of applying a living medical product.
In situ tissue engineering
Recently, in situ tissue engineering was proposed as a route to create living heart valves inside the body (Fig. 1 B, C). In this innovative approach costly and complex tissue culture is omitted and the body itself is used as bioreactor. Cell-free valvular shaped scaffolds are implanted at the site of destination where they gradually transform into living heart valves by recruiting cells from the bloodstream. Both synthetic and biological scaffolds can be used, provided that they can function as a load-bearing heart valve upon implantation and adequately guide and control neo-tissue formation. Contrary to in vitro tissue engineering, the technology is compatible with current regulation for medical devices and offers off-the-shelf availability at substantially reduced costs.
Collaboration
While this technology would constitute a simple and clinically attractive procedure, starting with the implantation of a non-living device, it requires the design of slow-degrading scaffolds that provide meticulous control over (selective) cell recruitment, cell fate, and load-bearing tissue formation. We propose the use of supramolecular polymers that can be fine-tuned with respect to degradation and mechanical properties, and functionalized with bioactive moieties to communicate with host cells in the course of tissue development2,3. It is hypothesized that neo-tissue formation follows the pathways of wound healing and to align this process interaction with inflammatory and immune cells, as well as balanced tissue formation in pace with scaffold degradation in the beating heart, is critical. To achieve the essential deep understanding of these interactions, multidisciplinary consortia of material scientists, engineers, clinicians and biologists have been established. Within larger (inter)national frameworks they collaborate with industrial partners and patient organizations to translate the technology to the clinic4-7.
Outlook
In situ tissue engineering holds great promise for the regeneration of damaged tissues and is foreseen to be valid for a broad range of applications. It provides the opportunity to create living tissue replacements that improve quality of life of many patients and that can outrange existing replacement therapies in terms of effectiveness, durability, availability, and costs. When successful, its simplicity and compliance with current regulatory protocols may favor a relatively smooth route to clinic.
1 Mol A, et al., Autologous human tissue-engineered heart valves: prospects for systemic application. Circulation 2006; 114(Suppl 1): I152-I158.
2 Bouten CVC, et al., Substrates for cardiovascular tissue engineering. Adv. Drug. Deliv. Rev. 2011; 63(4-5): 221-241.
3 Dankers PYW, et al., A modular and supramolecular approach to bioactive scaffolds for tissue engineering. Nat. Mater. 2005; 4: 568-574.
5 www.youtube.com/watch?v=oe_dmCbk7OY
7 http://ec.europa.eu/research/horizon2020/pdf/projects/lifevalve.pdf
Prof. Dr. Carlijn Bouten
Department of Biomedical Engineering & Institute for Complex Molecular Systems
Eindhoven University of Technology
Tel: +31 40 247 3006