Here, M. Danner and R.M Winglee from Department of Earth and Space Sciences at the University of Washington, share their expertise on the morphology of impact craters from shaped penetrators
The craters created by the shaped penetrator systems developed have a morphology that can be significantly different from that of natural craters. Typical craters produced by blunt or rounded impactors are wider than they are deep, giving the craters the classic bowl shape. The reason is that when on impact, blunt impactors compress the ground below it and if it has sufficient energy, breaks the ground up into small fragments. Because these fragments can more easily propagate radially, the crater is wider than it is deep, disrupting on average an area eight to 16 times larger than the impactor itself. (1)
Penetrators
In comparison, the average crater morphology from the shaped penetrators, shown in Figure 1, tends towards inverting the usual shape. The penetrators being used for sample return are hollow in the centre, allowing the ejected material to move vertically as opposed to radially. Instead of the shallow, wide craters typically seen at these impact speeds, the shaped penetrators are creating deep, narrow craters. The distinctive ejecta rim of typical simple craters isn’t seen with the shaped penetrator craters; the ejecta instead is columnized and ejected further from the impact site, not allowing for the accumulation of ejecta around the rim of the crater.
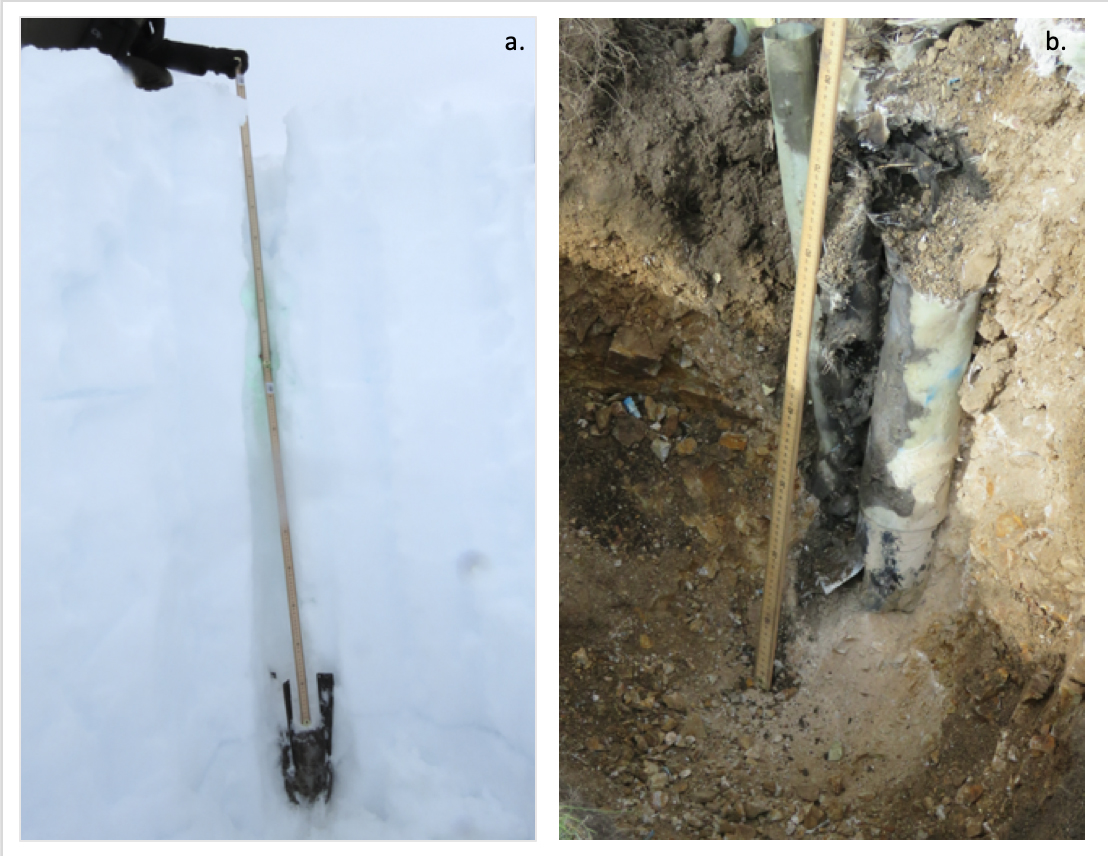
The overall characteristics of the crater are dependent on the hardness of the impacted material, speed of the penetrator, and diameter for penetrator. Due to these dependencies, the depth of the crater can be controlled by the penetrator and its speed. As shown in Figure 1, when impacting firn (seasonally compacted snow) the impactors, even without a powered descent (at ~80 m/s), excavated up to 1.7 metres into the surface. This is while maintaining an exceedingly narrow crater peaking at two times the impactor diameter at the surface, narrowing to the impactor diameter for the remainder of the depth. Into rock, such as granite with a roughly 10cm cover of alluvium, craters tended towards 1-1.2 metres in-depth, with a maximum diameter of 0.75 metres for speeds of 200-300 m/s.
Impacts into larger depths of alluvium exhibited much deeper craters, as seen in Part A of Figure 2. High power impacts into the loose sediment consistently showed 2 or more metre depths, minimal surficial cratering, and distinctive, well-formed “chutes” where the penetrator removed material. When a flared braking geometry was implemented on the penetrator, the depths of the penetrators drastically reduced to 0.5 metre scales, with a larger, more pronounced surficial cratering, as seen in Part B of Figure 2.
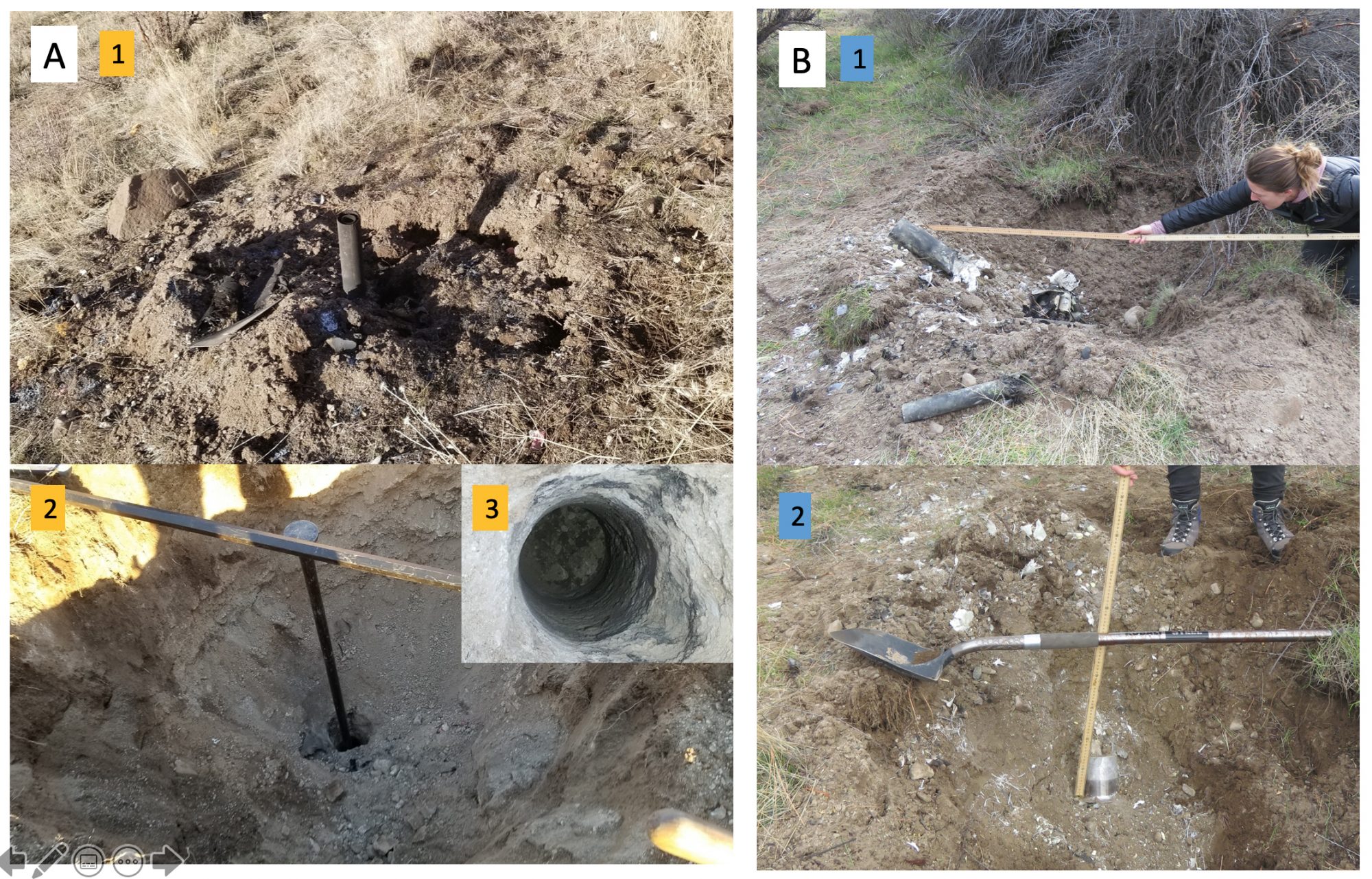
Figure 2. Part A – 4-inch diameter penetrator: 1) The crater pre-exhumation, 2) Mid-exhumation of the crater, 3) A view of the “chute” that was created by the impactor, which the breaker bar is inserted into in portion 2. Part B – 4-inch diameter penetrator with braking system: 1)
Pre-exhumation crater with scale, 2) Exhumed penetrator at a half metre depth.
Supersonic impacts
Upon reflection of the data, impactors which are hollow in the centre all have craters exhibiting the uniquely inverted crater morphology. Impacts at supersonic speeds did show a reduction in-depth and a broadening of the impact crater, though these impacts were also associated with impacts into hard rock. This leads to the following two controlling theories. First, craters from subsonic impacts have the smallest radial extend while cratering for supersonic impacts appear to have increased lateral extent, though this effect is unlikely to be relevant on airless solar system objects. The second theory concerns the material properties of the impacted material such as hardness, cohesion, and cleavage, which could very well be taking over in controlling the crater morphology in our impacts. It’s possible that the increased hardness, for example, was able to disperse the impact energy more similarly to a typical impactor. Supersonic impacts into roughly 10 metres of fine-grain alluvium or other non-consolidants results in 2 to 3 metre deep craters with no radial transmission, which exhibits a crater shape more reminiscent of a cookie cutter.
With a deeper understanding of the material properties controlling the crater morphology, these landing/sampling techniques develop an additional possibility: using the morphologies of these craters in extraplanetary surfaces to better understand the hardness, cleavage, or consolidation/layering of surfaces previously only known by remote sensing. Not only could the primary sample be utilised, but the landing technique itself will be able to teach us more about the impacted surface, whether it’s a comment, icy moon, or asteroid. Further work is currently underway analysing the microscopic effects of the impact force through the materials.
Reference
(1) David W. Hughes, The approximate ratios between the diameters of terrestrial impact craters and the causative incident asteroids, Monthly Notices of the Royal Astronomical Society, Volume 338, Issue 4, February 2003, Pages 999–1003, https://doi.org/10.1046/j.1365-8711.2003.06157.x
Please note: This is a commercial profile