Dr Stefan H. Bossmann and Dr Christopher T. Culbertson, Professors of Chemistry at Kansas State University, explain why microfluidic devices are in their view, the future
Microfluidics is all about controlling the flow of liquids on the micro-scale. In microfluidics, tiny channels are etched onto circuit boards, smaller than a human fingernail, through which minuscule volumes of chemicals and other liquids can flow.
One use of this technology is for everyday inkjet printers, where the channels help carefully control where the ink is sprayed in the printing process. These channels can also be merged, allowing two separate chemicals to mix and react, which is why some of these microfluidic devices are sometimes known as the ‘lab-on-a-chip’.
This team of investigators is led by Dr Christopher T. Culbertson and Dr Stefan H. Bossmann at Kansas State University. We are very excited by some of the possibilities that microfluidics and the lab-on-a-chip offer. In our highly interdisciplinary and collaborative project, our teams are working together to develop this technology into a miniature analysis lab. Our technology will, for the first time, offer profiling in a timely manner so that it could be used for “point-of-care-devices” (POC) capable of:
- Detecting the onset of a disease (e.g. cancer in a group of risk patients (BRCA1,2 or other mutations) or asthma/chronic obstructive pulmonary disease (COPD));
- Detecting the progress versus regress of a disease during treatment (e.g. chemotherapy);
- Detecting the recurrence of a disease (e.g. solid tumours versus other inflammatory diseases) and;
- Precision pain management.
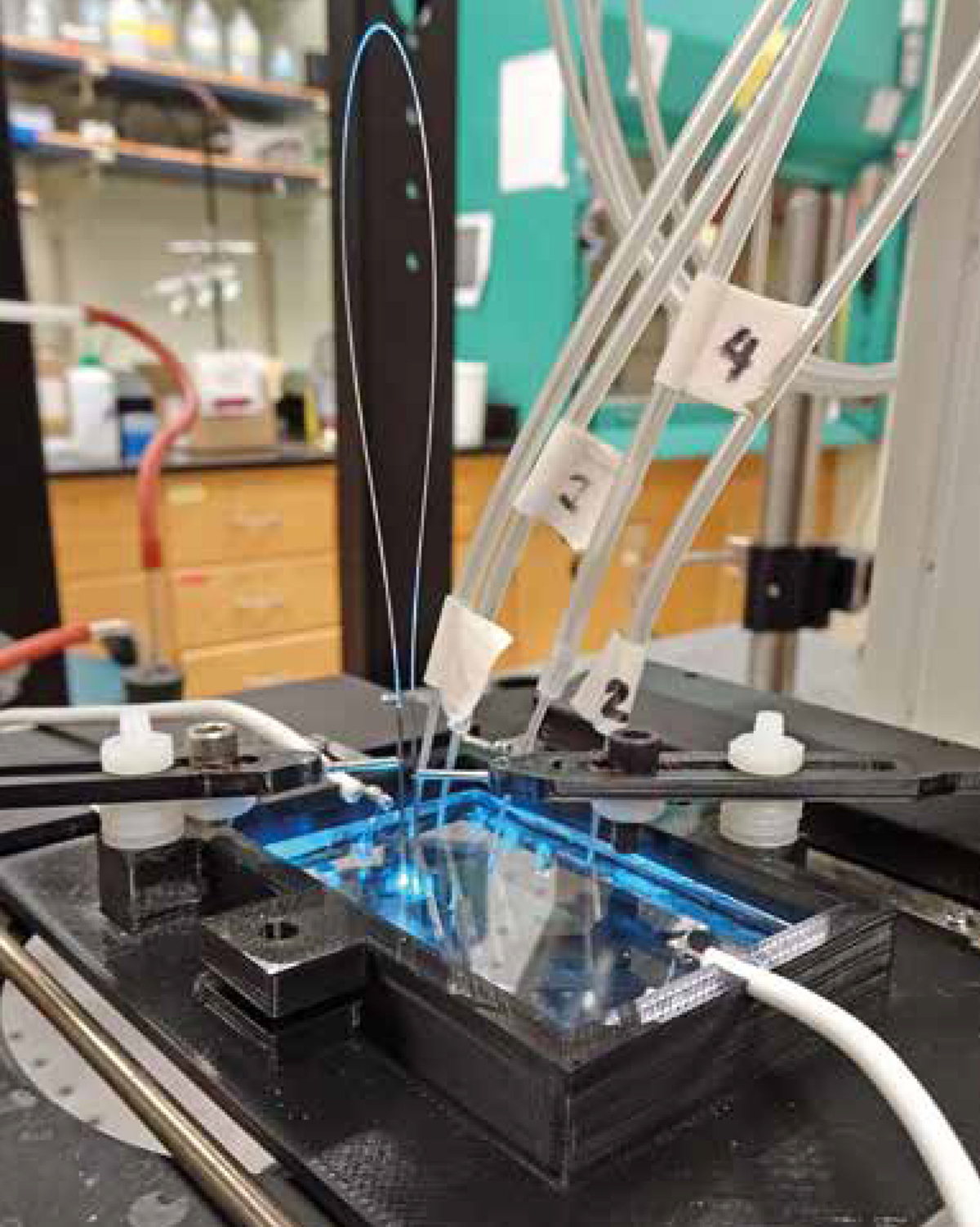
Our team is constantly designing new markers for reporting cell activities, such as key metabolic enzymes, proteolytic profiles, kinase network analysis and epigenetic reporting. Many of these markers are either designer peptides or contain designer peptides as functional elements.
Seeing cells
Microfluidic devices are inherently well-suited to looking at biological processes, as the micrometre channel size conveniently corresponds to the size of cells. Most cells are between 1 – 100 micrometres, with a human hair being approximately 60 micrometres thick. This means that the channels can not only be used to provide a highly controlled environment for cell growth, that is often more effective than a human-scale lab, but also to separate out different cells of different sizes.
We have already succeeded at using optical fibres to integrate this light detection technology onto a lab-on-a-chip. What is unique about our design and project is the off-chip placement of the optical fibre bridge: this means the chip design is not further complicated by the inclusion of the fibre. One of the big challenges with microfluidic devices is in their design; making components on such a small scale is difficult to do reliably and inexpensively, so this is a key advantage of our design.
Another unique feature of our project is creating a microfluidic device with multiple detection and excitation spots to detect the sample of interest, while still using only one laser and detector. The motivation behind this is to increase the versatility and capabilities of the device. Now with the integration of the optical fibres, they can detect the intact cell before the breakdown of the cell membrane, as well as the components from the cell after it is lysed.
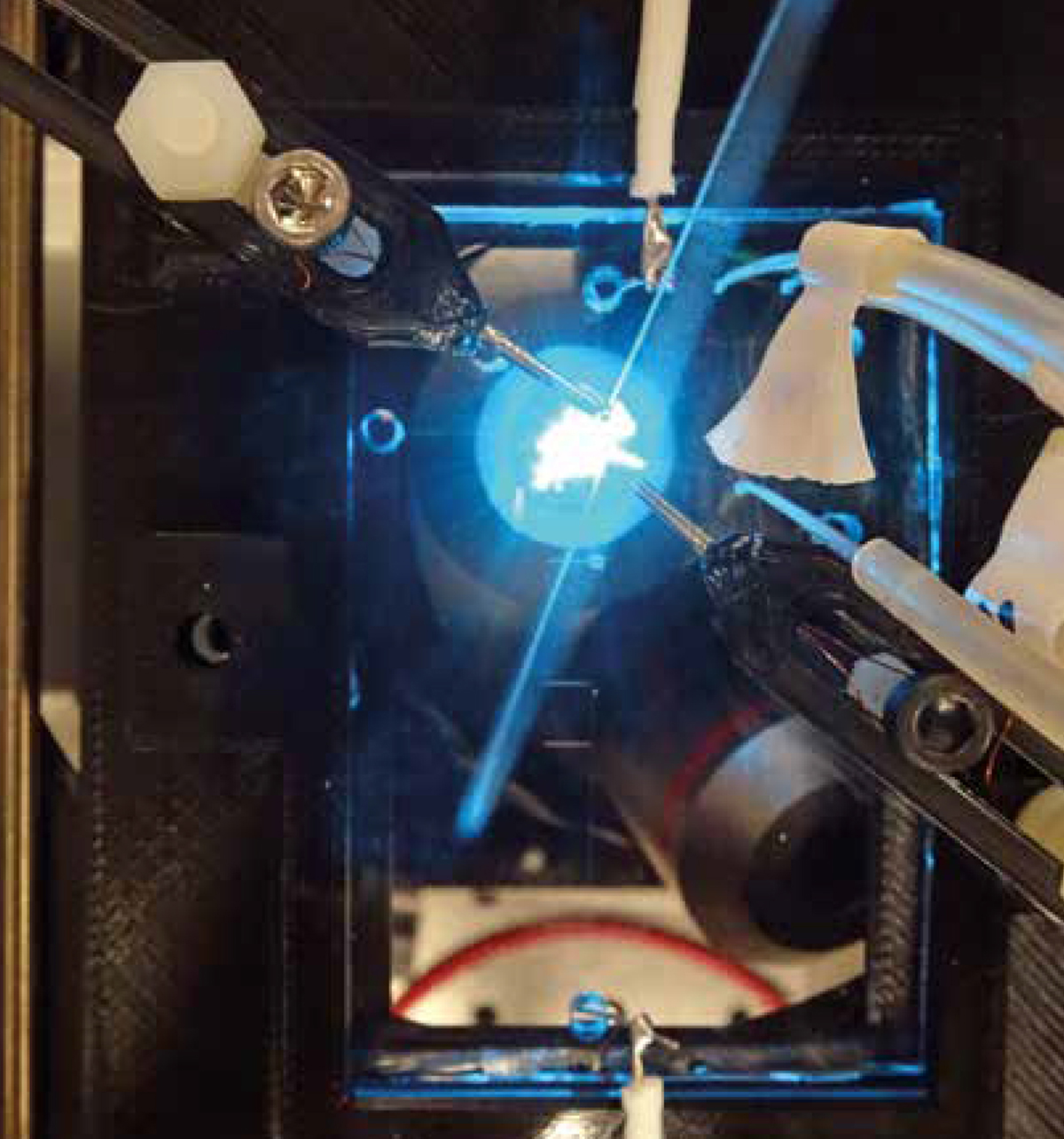
Each of the excitation spots on the microchip is like a viewing window for the cell’s activities, so the greater the number of spots you have, the greater the amount of information you can obtain. With more information, it becomes possible to better understand exactly how diseases lead to deformation and destruction of the cell.
Counting lines
We want to go beyond just being able to image and identify cells. This work involves designing very bright markers, so when the cells bind a chemical marker that glows after it absorbs light from a laser, this emitted light from the cell is sufficiently intense that a single molecule in a single cell can be detected. These markers also have to be rapidly taken up by the cell so that the detection can be done in ‘real time’. This is important if this device will be used to reduce patient diagnosis times.
This will be achieved by combining ultrabright fluorescent dyes or quantum dots with state-of-the-art peptide design, which enables the uptake and transport of markers to cellular targets (e.g. mitochondria or nucleus) within minutes. A large number of enzyme markers that can be monitored will allow for the detection of many possible diseases.
The work combining optical fibres with microfluidic devices will open up many new possibilities in understanding diseases at the cellular level and more tools for cell imaging and diagnosis. All of this is an important part in the development of lab-on-a-chip technology for making rapid, handheld diagnostic devices a routine part of healthcare.
Figure 2: Two 3-D printed micromanipulators are used to align the fibre with the microfluidic channels and the laser light emitted from a microscope objective
Prof Dr Stefan H. Bossmann
University Distinguished Professor
Kansas State University
Tel: +1 785 532 6817
sbossman@ksu.edu
www.k-state.edu/chem/people/grad-faculty/bossmann/MicrofluidicWelcome.html
www.twitter.com/BossmannDr
Prof Dr Christopher T. Culbertson
Associate Dean for Research
College of Arts & Sciences
Department of Chemistry
311 King Hall
Manhattan, KS 66506
culbert@ksu.edu
Tel: +1 785 532 6685
*Please note: This is a commercial profile