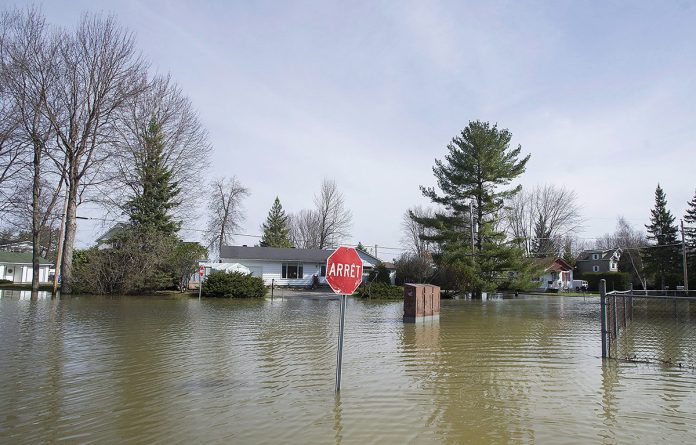
Professor René LAPRISE, ESCER Centre for the Study and Simulation of Regional-Scale Climate, discusses the recent trend in climate-related catastrophes
The globe has just experienced the hottest summer in 2019 since the start of measurements, with a temperature anomaly of 0.55°C compared to the average for 1981-2010, warmer by 1°C than the 20th Century average. Record-breaking heat waves have scorched Belgium, Netherland, Luxembourg, France, Germany, United Kingdom and Scandinavia. Never over the past 2000 years have temperatures increased as rapidly and as extensively as they did in recent decades. Scientific studies clearly demonstrate that this global warming trend is caused by the prolonged emissions of greenhouse gases (GHG), especially carbon dioxide produced by burning fossil fuel for energy.
The global warming is accompanied by several changes in local weather. The occurrence of extreme weather events and hydrometeorological hazards has greatly accelerated since the 50’s, causing losses of lives, property damages and environmental degradation. More than 23 million people were forced from their homes as a result of weather-related events during 2016. The 2017 hurricane season was the most devastating ever in the Atlantic, with no less than three high-impact storms – Harvey, Irma and Maria, causing damage total of 295 billion U.S.$. The Southern Québec region of Canada alone has suffered no less than four record-breaking spring floods in recent years – 2011, 2014, 2017 and 2019. The impacts of storms involve a combination of factors, such as increased exposition and vulnerability, but climate change does contribute. Why should global warming affect local storms?
Water vapour feedback on storms
Storms are energetic atmospheric eddies that occur over a wide range of spatial and temporal scales. But even the largest weather systems contain fine-scale structures, such as fronts, where most intense precipitation occurs.
Water vapour is a gas, invisible to the naked eye, third in abundance in the atmosphere where its concentration varies greatly. The water vapour holding capacity of the atmosphere varies with temperature, following a thermodynamic law known as the Clausius-Clapeyron equation. When air parcels undergo upward motion in storms, they cool under the effect of pressure drop and volume expansion. Below some temperature, saturation is reached, and water vapour begins to condensate into droplets or ice crystals, seen as clouds, which will eventually fall as precipitation. Water vapour condensing to cloud in a storm involves large amount of heat, 2.5 million Joules per kg (700 W h per kg). This condensational heating constitutes an important positive feedback in storm dynamics: the stronger a storm, the stronger the vertical motion, the more important the heat release that fuels storms. Hence precipitation is both a by-product of storms that can cause hydrometeorological hazards, and an important positive feedback mechanism contributing to strengthening storms.
A warmer atmosphere is able to hold more water vapour. It has been estimated that for every degree of global warming, a 7% increase of water vapour can be expected. Hence for a global warming of 3 to 6°C expected for the end of the century, a 21 to 42% increase of water vapour can be expected on average. This implies that, as the Earth system warms as a result of the continual release of GHG due to the combustion of fossil fuel, storms threaten to become stronger, and hence more destructive. But where and by how much?
Future weather simulators
The analysis of weather observations informs us of the current state of the climate and its recent evolution. But how can we foretell the future of climate? This is where computer simulations become handy tools. In the same way that flight simulators serve to train pilots without risks, virtual weather simulators – also known as climate models (CM), are used to make projections of future weather conditions for several years ahead, subject to altered conditions such as enhanced GHG concentration in the atmosphere.
The behaviour and interactions of the components of the Earth system – atmosphere, oceans, sea ice, land surface, vegetation and snow, follow the laws of physics. These complex nonlinear coupled partial differential equations are discretized in the horizontal and vertical dimensions on a three-dimensional grid that cover the Earth’s surface, from the depth of the oceans to the top of the atmosphere.
The calculations being carried over several decades of simulated time require humongous computational resources, several months of calculations on even the fastest supercomputers. The computational burden is function in particular of the number of grid points, hence there are two options: either spreading them to cover the entire Earth surface (global CM, GCM) or concentrating them over a limited region of interest (regional CM, RCM). GCM simulations with meshes of the order of 100 km or even coarser only resolve the largest storms, with a blurred view of the fine scales such as fronts and rain bands that cannot adequately be resolved.
RCM meshes have constantly been refined, and today simulations are possible using meshes of 2 to 4 km.
Such high-resolution grids can resolve frontal rain bands, convective processes responsible for thunderstorms, and mountain and valley winds. The so-called convection-permitting RCM (CP-RCM) account for the positive feedback of precipitation and storm dynamics, and exhibit much improved simulation of the intensity, frequency and distribution of heavy precipitations.
Responsible governments must make informed decisions advised by the best scientific knowledge and using the best tools to reduce vulnerability and increase resilience. CP-RCM are an essential tool to anticipate weather-related hazards that will face humanity over the forthcoming decades under the threat of global warming.
Acknowledgements: This work was funded by the “Marine Environmental Observation, Prediction and Response” (MEOPAR) project and the Discovery Grants Program of the Natural Sciences and Engineering Research Council of Canada (NSERC).
Please note: This is a commercial profile