Professor Ulrich Flögel, Heinrich Heine University Düsseldorf, details to us how to reveal the functional impact of myoglobin redox homeostasis by cardiac magnetic resonance
With magnetic resonance techniques, not only anatomical images of the inside of the body can be acquired, but by dedicated techniques also foci of inflammation or small molecular compounds can be determined in individual organs, such as the heart, without radiation exposure. Thereby, important issues concerning cardiac metabolism can non-invasively be addressed, in particular, high energy phosphate turnover as well as substrate utilisation and lipid homeostasis. Beyond this, special proteins like myoglobin can be assessed by 1H magnetic resonance spectroscopy (MRS), which provides the opportunity to address also oxygen tension and redox state of the heart.
What is Myoglobin?
Myoglobin is a small hemoprotein found in the cytoplasm of skeletal and heart muscle in quite high concentrations. Myoglobin-containing muscles are red; if the myoglobin content is reduced, as in poultry meat, or if myoglobin is lacking completely due to genetic manipulation, the muscle fibres look much paler. Similar to its molecular relative haemoglobin, which manages the O2 transport from the lung to the cells, myoglobin reversibly binds O2 but with a six-fold higher affinity than the – with the bloodstream circulating – haemoglobin. Thereby, it can easily take over the haemoglobin-provided O2 from the capillaries and facilitate O2 transport from the blood to mitochondria during periods of increased metabolic activity or serve as an O2 reservoir under hypoxic conditions. The latter is, in particular, supported by the up to tenfold higher myoglobin content in muscles of diving vertebrates, such as dolphins and whales.
Beyond the well-known O2-binding properties of myoglobin, within the last few decades, the field of globin biology was strongly invigorated, particularly by the generation of gene-deficient mutants but also by the discovery of two new members of the globin family, cytoglobin and neuroglobin. Based on this recent work, the role of myoglobin in muscle physiology has been reassessed and its scope of function has been considerably extended beyond oxygen storage and delivery to also include a role as an important scavenger of nitric oxide (NO) – an important signaling molecule that is also involved in nitrosative stress. The key to all of these investigations was the non-invasive measurement of myoglobin in the heart by 1H MRS.
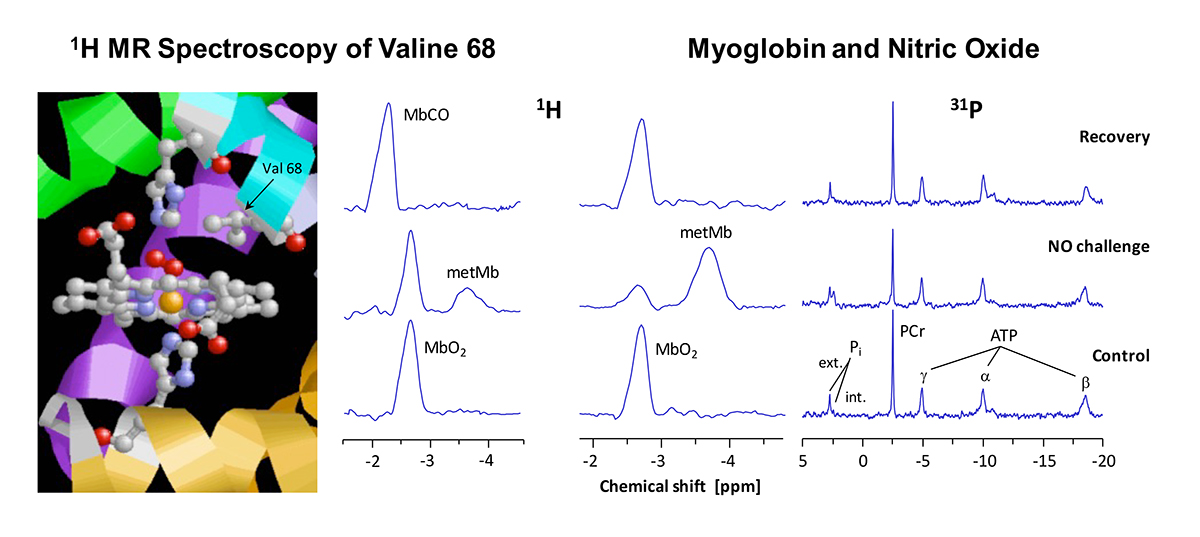
The figure shows in detail the structure of myoglobin’s heme group. O2 binds to the central iron atom (orange) which is surrounded by four nitrogen atoms (blue) in the plane of the porphyrin ring. The lower coordination site of the iron is occupied by another nitrogen (of the “proximal” histidine, His93 or F8), whereas the O2 bond takes place above the ring plan. The binding of O2 is additionally stabilised by a hydrogen bridge bond to the “distal” histidine (His64 or E7). In the immediate neighbourhood, one can recognise the methyl groups of valine 68 (E11), whose protons can be used as reporter nuclei of the current situation at the heme.
When the heart tissue is adequately supplied with O2, myoglobin – owing to its high O2 affinity – is completely oxygenated (MbO2), and the methyl protons of the valine can be detected at a chemical shift of -2.7 ppm by 1H MRS of the heart (bottom). Only at a mismatch between O2 supply and consumption, myoglobin gets deoxygenated which is reflected in an intensity decrease of the MbO2 signal. In that, the magnitude of this signal permits an assessment of the myocardial O2 supply. Making use of a simple mathematical transformation, the intensity of MbO2 signal can be utilised to calculate the intracellular O2 partial pressure of the cardiomyocytes – a quantity, which is very difficult to access with other methods.
The oxygenation level is, however, not the only parameter, which affects the signal of the valine protons in 1H MRS. Generally spoken, each change of the chemical or electrical environment at the heme results in an alteration of the signal’s intensity or position. For example, when the poisonous carbon monoxide (CO) ejects the bound O2 due to its higher affinity to the heme iron, the formed MbCO is reflected in the spectrum by a signal at -2.3 ppm (top). Besides a ligand exchange, the oxidation state of the iron also affects the proton signal: if the iron in the centre of myoglobin’s heme loses an electron and converts from the normally divalent to the trivalent state – generally termed as metmyoglobin (metMb) – the fraction of the generated metMb can be unambiguously detected by its signal at -3.8 ppm (middle).
Monitoring the protons
Thus, monitoring the protons of the valine 68 by 1H MRS as reporter nuclei of the electronic situation at the heme group yields versatile information about oxygenation, ligands and the redox state of cardiac myoglobin. This is further illustrated on the right showing 1H and 31P MRS of a heart exposed to an acute NO challenge. Under NO infusion, a new signal at -3.8 ppm was detected, that can be unequivocally assigned to metMb (see above), while concomitantly, a decrease of the MbO2 signal at -2.7 ppm was observed (middle). 31P MRS indicated at the same time a serious disturbance in the cardiac energy state with decreased phosphocreatine (PCr) and increased inorganic phosphate levels (Pi). After cessation of the NO challenge, MbO2 and energy state were found to be rapidly regenerated (top), suggesting the presence of a robust metMb reductase activity. Thus, the metMb signal became detectable only when cardiac metMb production from MbO2 and NO exceeded the capacity of metMb reductase to reconvert metMb into Mb.
Experiments
Further experiments about the dose-dependent effect of NO on function and energetics of normal and myoglobin-deficient (Mb-/-) hearts revealed, that exactly in the NO concentration range that caused the formation of metMb, Mb-/- hearts were more sensitive to NO, in that depression of contractile as well as energetic parameters were more pronounced when compared with normal hearts.
Results
The described results can easily be reconciled with well-known chemical reactions of myoglobin and NO. However, although the individual steps have been exhaustively studied in vitro, it was not clear that together, they constitute a metabolic cycle that has functional relevance in cardiac NO homeostasis in vivo. Obviously, the reaction of MbO2 + NO → metMb + nitrate represents a “chemical hoover”, which takes care that even during enhanced NO formation cytosolic NO levels are kept extremely low, and by this protects the respiratory chain from detrimental effects of the reactive NO species.
Taken together, these experiments demonstrate that myoglobin serves as an important cytoplasmic scavenger of bioactive NO, and thereby, as a barrier for protection of the heart against nitrosative stress.
Please note: This is a commercial profile