Dr Samuel Langendorf, Los Alamos National Laboratory, details some interesting results in the area of plasma jet driven magneto-inertial fusion (PJMIF) and his thoughts on the race for fusion energy
In the five previous Open Access Government articles, we have described the plight of controlled nuclear fusion research over the past decades and have chronicled the progress of our work on an innovative new approach called plasma-jet-driven magneto-inertial fusion (PJMIF.)1 In this article, we present current conclusions and directions of our research in PJMIF and remark on the changing landscape of fusion energy research, driven increasingly by private investment, public-private collaboration and growing global alarm at the rapid onset of anthropogenic climate change.
We are exploring the physics of PJMIF at the Plasma Liner Experiment (PLX) at Los Alamos National Laboratory. One of the most important questions that PLX is designed to explore is: can a smooth, supersonic, spherically imploding plasma liner actually be formed from the merging of discrete plasma jets? Standard computer simulation techniques for dense plasmas, based on hydrodynamics, suggest that the formation of shock waves between merging jets may lead to significant density perturbations in the formed liner, making it difficult or perhaps even impossible, to form the desired smooth spherical liner. However, simulations disagree2,3 on the sharpness and magnitude of the perturbations predicted to be formed by the shocks, with little experimental data available in the intermediate-density regime that PJMIF targets. At PLX, we performed experiments to obtain benchmarking results for these models in a relevant parameter regime.
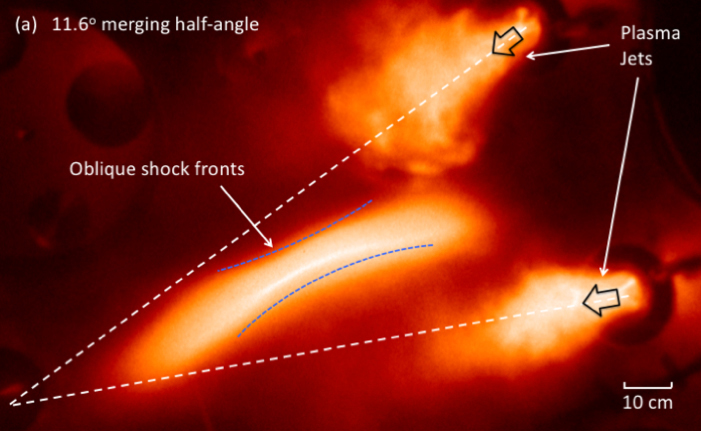
To explore the physical processes underway in the jet merging, we used the coaxial plasma guns designed and built by HyperJet Fusion Corporation (detailed in several of the preceding articles in this series) to fire supersonic plasma jets and collide them at an oblique angle. These experiments are conducted at reduced energy levels to those estimated to be required to produce breakeven fusion, but allow the physics to be studied at a greatly reduced cost. By varying the angle of the jet merging, we can study the effect of the jet relative velocity on the merging processes. Example fast-camera images of the plasma jet merging are shown in Figure 1.
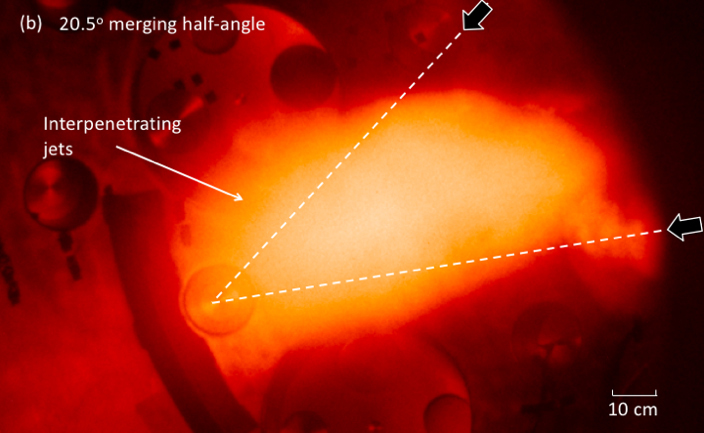
The observations indicate there is more to the story of the merging plasma jets than the hydrodynamic prediction alone would indicate. For example, in Figure 1a, jets collide at a relatively shallow angle and low (but still supersonic) relative velocity and shocks form in accordance with hydrodynamics. At steeper merging angle, however, shown in Figure 1b, rather than a stronger shock wave as predicted by hydrodynamics for the higher relative velocity, a smoothed and diffuse merging structure is observed. We find this result to be due to significant interpenetration of the jets occurring on the merging length scale, preventing the formation of a sharp density perturbation as would occur in a hydrodynamic shock.
With benchmark data such as these now available from PLX, we can look forward to projected results at fusion-relevant scale. Such plasma liners would be denser by orders of magnitude than the PLX experiments, raising the question of whether the smoothing effects of interpenetration observed at PLX can be sustained at a fusion-relevant energy scale. Looking at plasma interpenetration theory, we project that similar smoothing will indeed be achievable if the liner is driven at a higher velocity, an encouraging result for the hope of utilising PJMIF as an effective fusion compression driver. These and other results from PLX are detailed in the current4,5 and upcoming publications.
Going forward, we are upgrading PLX to perform hemispherical and ultimately fully spherical experiments, with a significantly increased number of plasma guns. At the time of this writing, 18 new plasma guns have been delivered by HyperJet Fusion Corp. and hemispherical and spherical experiments are planned with up to 36 guns before the end of the calendar year. Such experiments will give important results on the density amplification and ram-pressure scaling achieved in spherically convergent geometry, information that is a key metric of the concept performance and needed to benchmark designs of a full-scale driver.
To conclude this article series, we recall the case made by Dr Scott Hsu in the first article in this series, which is that fusion energy development has been held in check in recent decades by two factors: a high ~$10 billion capital cost of modern facilities and a lack of urgency and public political will in fusion energy development. Today, three years later, the first factor may be finally showing signs of weakness due to aggressive new scientific approaches aimed to achieve economical fusion power at a much cheaper development cost than the current worldwide tokamak-based path. These approaches include PJMIF and other exciting projects6,7 supported by the ARPA-E ALPHA program, as well as new approaches in magnetic confinement fusion that seek to use high-temperature superconducting magnets to achieve a productive tokamak reactor at significantly reduced size and facility capital cost8. The second factor, lack of urgency will, may also be weakening as the effects of climate change grow ever more severe and apparent. Private risk capital and philanthropic interests have moved to support fusion at unprecedented levels and the government has responded with new programmes such as the DOE Office of Fusion Energy Sciences INFUSE program to complement that effort. Whether these approaches will be enough to achieve practical fusion power in time to impact climate change, only time will tell.
References
- Thio, YC Francis, et al. Fusion Science and Technology (2019): 1-18.
- Shih, Wen, et al. Physics of Plasmas 26.3 (2019): 032704.
- Thompson, Seth, and Jason Cassibry. Physics of Plasmas 26.5 (2019): 052701.
- Langendorf, Samuel J., et al. Physical review letters 121.18 (2018): 185001.
- Langendorf, Samuel J., et al. arXiv:1905.02276 (2019).
- Nehl, C. L., et al. arXiv:1907.09921 (2019).
- Zhang, Y., et al. Physical review letters 122.13 (2019): 135001.
- Greenwald, Martin. Joule 3.5 (2019): 1175-1179.
Please note: This is a commercial profile