John Greenwood PhD FRCPath and Stephen E. Moss PhD from UCL Institute of Ophthalmology discuss the vasculature in health and disease, a major focus of their research
The human vasculature is an exquisite hierarchical system that is responsible for the delivery of blood to every organ of the body. Adult humans are estimated to have almost 100,000 km of blood vessels, with the bulk of this impressive figure coming from small microvessels that permeate throughout the tissue where they exchange gases, nutrients, waste products and immune cells with the local environment.
During development, blood vessels grow through angiogenesis to serve the needs of the expanding tissue, a process that has been studied extensively and that has illuminated the key signalling molecules and pathways involved. New vessels also undergo a process of stabilisation and maturation that confers physiological function according to their hierarchical position and the requirements of the organ in which they lie. Once mature they provide a selective and regulatory barrier between the circulating blood and the tissue.
The vasculature has a remarkable capacity to maintain its function throughout life but, not surprisingly given its ubiquitous presence, it is also involved in many major diseases. Vascular dysfunction may manifest in a number of different ways including barrier breakdown leading to fluid leakage (oedema) and bleeding (haemorrhage), vessel degeneration or blockage (stroke) resulting in oxygen deprivation (ischaemia) and the uncontrolled growth of abnormal new vessels (neovascularisation). These defects may be the primary cause of the disease, or a secondary consequence of another disease process, but in both cases vessel dysfunction is highly damaging.
New vessel formation in disease
Blood vessels that grow in diseased tissue are nearly always abnormal; forming a chaotic vascular network that leaks fluid, is susceptible to rupture, and poorly perfused, characteristics that contribute to progression of the particular disease. Archetypal amongst these conditions are the solid cancers and retinal diseases including neovascular (or wet) age-related macular degeneration (nAMD) and proliferative diabetic retinopathy (PDR).
It has been known for many decades that solid tumours are able to stimulate new vessel growth to support their expansion. Indeed, it has been postulated that cancers cannot grow much larger than a few millimetres diameter without this happening (Folkman 1971). Whilst this view has been somewhat modified in recent years, as some cancers can utilise existing vessels, this general principle still holds true. For most of the last century, therefore, the prevailing opinion has been that if new vessel growth could be inhibited then this would constrain tumourigenesis.
As mentioned above, a great deal is known about the factors responsible for driving developmental angiogenesis and it is clear that the same key factors are also responsible for mediating neovascularisation in cancer. Principle among these is vascular endothelial growth factor (VEGF) and its signalling partners that are induced in the absence of oxygen (Apte et al 2019). Some 15 years ago a biologic (antibody – Bevacizumab) targeting VEGF underwent clinical trials in cancer and its use has had some success in increasing progression-free survival in certain cancers, although with little impact on overall survival rate.
Nevertheless, in a wonderful example of opportunism, bevacizumab was demonstrated to be highly effective in blocking new vessel growth in sight-threatening ocular diseases, most notably nAMD, and more recently in PDR. Nonetheless, many patients remain refractory to VEGF axis blockade illustrating a continued unmet clinical need.
What has been highlighted by these vascular targeted approaches is that there is still much that we don’t understand about pathological neovascularisation.
For example, angiogenesis can escape from the influence of the prototypical drivers and, in different disease settings, control of vessel growth is clearly finely nuanced. With a growing appreciation of the role the vasculature plays in human disease it is therefore critical that new targeted therapies are developed to treat those conditions that remain non-responsive.
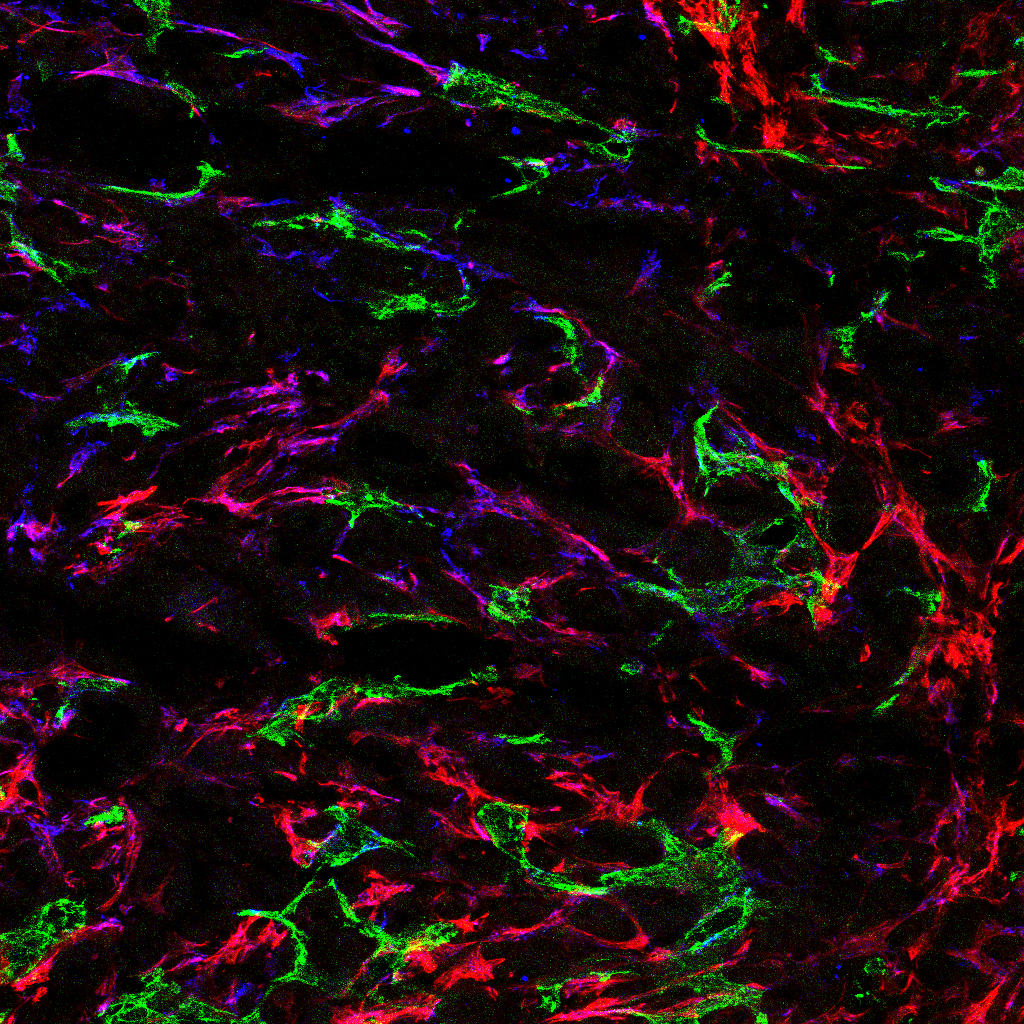
Left image: Blood vessels (green) and vessel support cells (mural cells – red and blue) in a model of pancreatic cancer. Right image: Abnormal vessels in model of retinal vascular disease.
Development of drugs that target new vessel growth
There continue to be advances in developing drugs that target the VEGF axis and related pathways, but other targets need to be identified and new therapies developed. Recently great interest has arisen surrounding the role of metabolism in regulating vessel growth (Potente & Carmeliet 2017). Perhaps not surprisingly, as in oxygen deprivation, paucity in the supply of essential metabolites is a trigger for new blood vessel growth. It is likely to be only a matter of time before new therapeutic approaches based on this knowledge will become mainstream.
In our own laboratory, work funded by the Medical Research Council (UK), the Wellcome Trust, the British Heart Foundation and University College London Technology Fund, led to the identification of a novel therapeutic target, leucine-rich alpha-2 glycoprotein-1 (LRG1) (Wang et al 2013). Unlike the VEGF axis, this secreted molecule is not involved in developmental angiogenesis but is only induced in diseased tissue where it corrupts the normal angiogenic process. This discovery may explain why blood vessels grow abnormally in disease.
Interestingly, in cancer there is a growing view that rather than destroy abnormal tumour vessels or inhibit their growth, it may be more appropriate to improve their function; a process termed vascular normalisation (Jain 2015). This is predicated on evidence that an inadequate blood supply is both pro-oncogenic and limits the delivery of therapeutics. In laboratory studies we have found that blocking LRG1 improves vascular function and the delivery of other tumour drugs. Based on our findings we have developed a therapeutic antibody (Magacizumab) and clinical trials in ocular neovascular disease and cancer are planned through the support of UCL Business and the formation of a spin-out company.
The future
Therapeutic targeting of the vasculature is likely to be of increasing importance in the treatment of many diseases and with personalised medicine and greater use of combinatorial drug approaches the outlook is promising. However, many obstacles lie ahead not least of which is the challenging funding landscape and the possible damage to cross-EU research threatened by Brexit. Laboratories throughout Europe have made and will if given adequate support, continue to make a substantial contribution to our knowledge in this field. Governments and funding agencies must, therefore, be prepared to invest long-term in both fundamental discovery science in this area as well as translational proof of concept research.
As it can take the best part of 15 years to go from target discovery to clinical trials long-term strategies are essential. It is recognised that the attrition rate along the road to delivering new treatments is high but the rewards, both in terms of health and wealth, are considerable. For example, the tens of millions of people worldwide diagnosed with cancer, nAMD and PDR each year will benefit, and with the market value of anti-VEGF therapies alone in these diseases currently ~$10 billion per annum it is incumbent on the European governments to ensure that there is adequate investment in this area.
References
Potente M, Carmeliet P. The Link Between Angiogenesis and Endothelial Metabolism. Annu Rev Physiol. 2017 Feb 10;79:43-66.
Apte RS, Chen DS, Ferrara N. VEGF in Signaling and Disease: Beyond Discovery and Development. Cell. 2019 Mar 7;176(6):1248-1264.
Folkman J. Tumor angiogenesis: therapeutic implications. N Engl J Med. 1971 Nov 18;285(21):1182-6.
Wang X, Abraham S, McKenzie JAG, Jeffs N, Swire M, Tripathi VB, Luhmann UFO, Lange CAK, Zhai Z, Arthur HM, Bainbridge J, Moss SE, Greenwood J. LRG1 promotes angiogenesis by modulating endothelial TGF-β signalling. Nature. 2013 Jul 18;499(7458):306-11.
Jain RK. Normalization of tumor vasculature: an emerging concept in antiangiogenic therapy. Science. 2005 Jan 7;307(5706):58-62.
Please note: This is a commercial profile
John Greenwood PhD FRCPath
Davson Professor of Biomedical Research
UCL Institute of Ophthalmology
Tel: +44 (0)207 608 6858
https://iris.ucl.ac.uk/iris/browse/profile?upi=JGREE74
Stephen E. Moss PhD
Ashton Professor of Biomedical Research
UCL Institute of Ophthalmology
Tel: +44 (0)207 608 6973