Jay Sibbitts, Prof Dr Stefan H. Bossmann and Prof Dr Christopher T. Culbertson, from Kansas State University, Department of Chemistry in the U.S., underline microfluidic detection of intracellular nitric oxide levels
Despite decades of research, diseases like glioblastoma, Alzheimer’s disease and Parkinson’s disease remain incurable. However, it is clear that changes in the inflammatory response in the brain play a central role in the progression of these diseases and, therefore, may serve as a potential route for treatment. During inflammation, immune cells not only attack the initial threat, but also recruit other nearby immune cells through signalling molecules and proteins.
Nitric oxide (NO) is an inflammatory signalling molecule that has been of interest for study not only as an indicator of inflammation in cells, but also because of its ability to inflict cellular damage under prolonged inflammation. NO is produced by a family of nitric oxide synthase enzymes (NOS): endothelial nitric oxide synthase (eNOS), neuronal nitric oxide synthase (nNOS) and inducible nitric oxide synthase (iNOS). NO exists in vivo as a dissolved free radical gas, with an unpaired electron on the nitrogen. Increased iNOS expression during inflammation can lead to excessive NO levels, resulting in detrimental effects like protein nitration, DNA damage and programmed cell death (Figure 1).
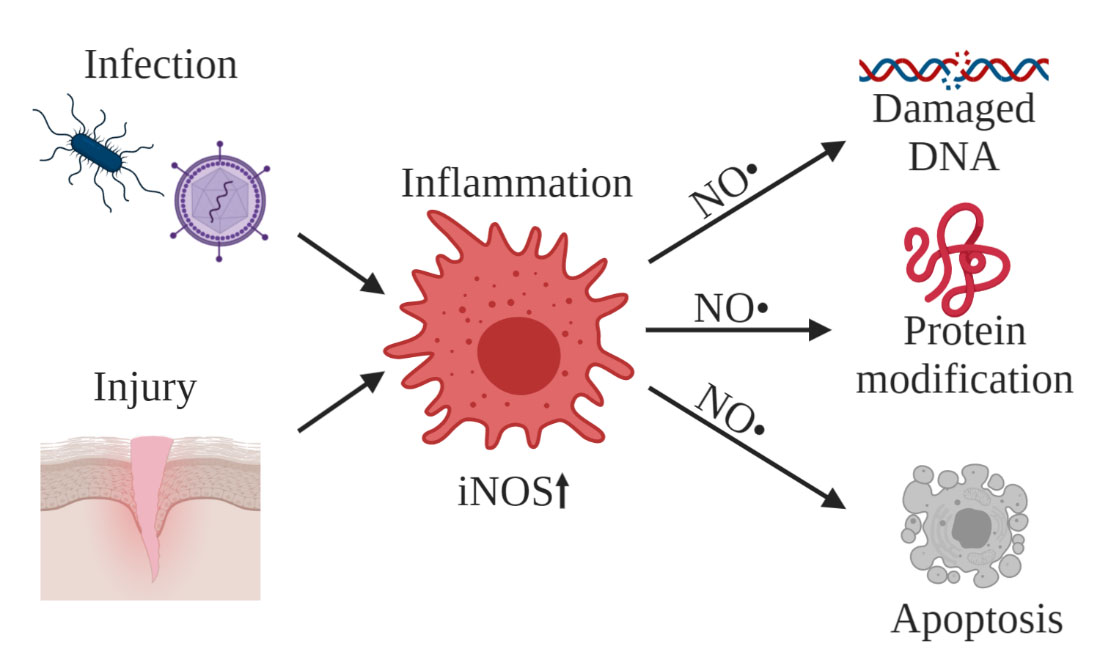
Measuring nitric oxide levels
Traditional techniques typically measure extracellular NO levels. NO levels can change rapidly. Accurately measuring NO produced within cells using traditional techniques is particularly challenging because of its short half-life (3-6s).1 Furthermore, NO production can vary widely within a population of cells, and measuring extracellular NO is not able to account for subpopulations of cells that may be producing excessive amounts.
A technique capable of rapidly measuring intracellular NO produced within individual cells may provide a clearer picture of the behaviour of immune cell populations over the course of the inflammatory response. To that end, the Culbertson research group has been working on the development of a high-throughput single-cell analysis microfluidic device that is capable of quantifying levels of intracellular analytes following fluorescent labelling or derivatization.
A 3D representation of the single-cell analysis device is shown in Figure 2. The design, fabrication and working principles of the device are discussed in our previous publications and were also briefly discussed in our previous profile.2 The device features continuous cell transport via on-chip pumping followed by automatic electrokinetic lysis of individual cells and ultimately electrophoretic separation and quantitation of intracellular analytes.
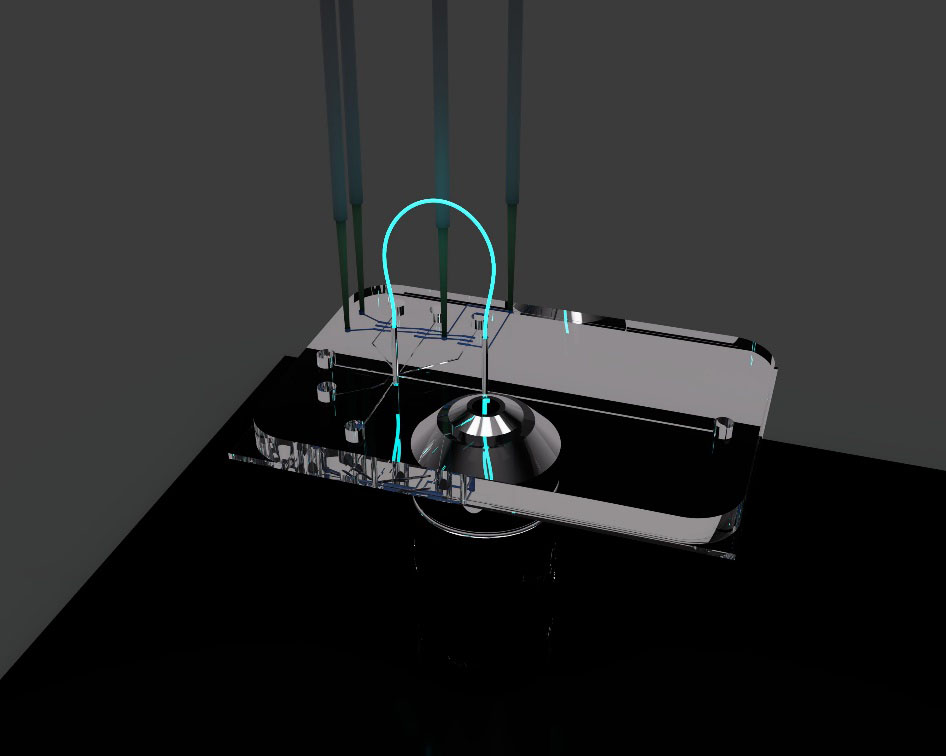
Nitric oxide analysis
For NO analysis, a diaminofluorescein derivative (4-amino-5-methylamino-2’,7’-difluorofluorescein diacetate), or DAF-FM DA, is used to selectively react with intracellular NO. Upon reaction with NO, non-fluorescent DAF-FM DA is derivatized to its strongly fluorescent triazole form (DAF-FM T). To account for variations in cell volume and dye uptake, cells are co-labelled with an internal standard, non-fluorescent 6-carboxyfluorescein diacetate (6-CFDA) which is modified inside the cell to fluorescent 6-carboxyfluorescein (6-CF). This means that for every cell there are two fluorescent peaks detected once the cell contents are electrophoretically separated.
In electrophoretic separations, peaks are identified by their characteristic migration times in a given set of separation conditions (e.g. buffer composition and electric field strength). To measure migration times, a second detection spot is incorporated in the device to detect cells just before they are lysed. This is done by integrating an optical fiber bridge that transmits a fluorescent signal from one end to the other. One end collects fluorescence emission from the intact cells as they pass underneath and the other directs that signal to the detection optics. This means that there are now three peaks detected for a single-cell: the intact cell signal, 6-CF, and DAF-FM T.
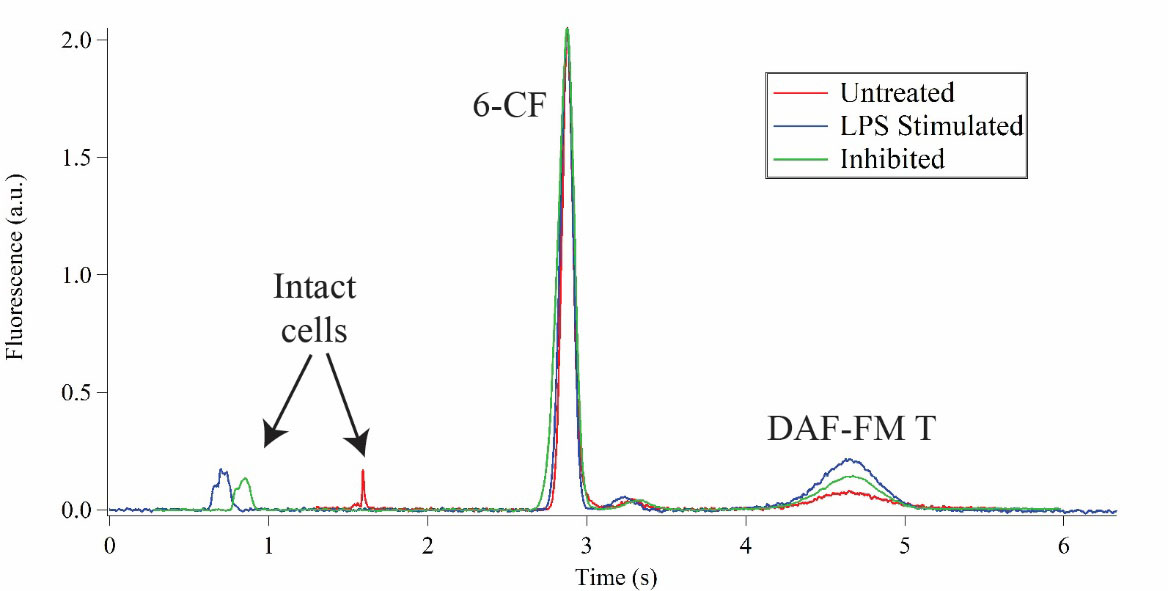
Beyond correctly identifying separated intracellular analytes, it is perhaps more important that our device can detect changes in NO levels in response to inflammatory stimulation, as well as inhibition of iNOS. To test our system’s capabilities for this application, we measured NO levels in immortalized T lymphocytes (Jurkat) to serve as a model immune cell. An inflammatory response was elicited using lipopolysaccharides (LPS) which mimics a bacterial infection. An increase in the peak area of the DAF-FM T peak relative to 6-CF indicates elevated NO production. To measure the effects of inhibition, we chose a potent and selective iNOS inhibitor, 1400W dihydrochloride. The cells were treated with both LPS and 1400W dihydrochloride followed by NO measurements. Representative single-cell measurements for untreated cells, stimulated cells and inhibited cells are shown in an overlay in Figure 3. Cells stimulated with LPS produced 2.2-fold more NO as compared to untreated cells, and cells treated with the inhibitor only increased NO production 1.2-fold.
Studying inflammatory response
Through this work, we have demonstrated that our system can be a valuable analytical tool for the study of the inflammatory response. Future efforts will be directed toward expanding the application of our device to other cell types, such as microglia. Microglia are the resident immune effector cells in the brain, and studying their behaviour under different inflammatory conditions could provide additional insights into understanding the nature of incurable brain diseases like glioblastoma, Alzheimer’s and Parkinson’s, among others.
References
1 Pacher, P.; Beckman, J. S.; Liaudet, L., Nitric oxide and peroxynitrite in health and disease. Physiol Rev 2007, 87 (1), 315-424.
2 Sibbitts, J.; Sadeghi, J.; Culbertson, C. T., Microelectrophoretic single-cell measurements with microfluidic devices. Methods Enzymol 2019, 628, 223-241.
Please note: This is a commercial profile
Contributor Details
Editor's Recommended Articles
-
Must Read >> Microfluidic devices: The future is here