Here, Professor Patricia Luis (1,2) from UCLouvain explains how integral CO2 capture is along with the production of sodium bicarbonate crystals in reducing global emissions
The current climate crisis highlights the essential necessity of changing people’s lifestyles in countries with more developed economies. Business as usual is not acceptable anymore, even if societal change is difficult. Besides this societal transformation, the industry must join efforts to adapt to new necessities and more restrictive energy use.
Emissions of carbon dioxide (CO2), a major contributor to global warming, must be also drastically reduced if climate neutrality is the objective by 2050 [1]. In this context, the use of fossil-based carbon become incoherent, but carbon is still a basic element in the production of base chemicals and polymers.
A new source of carbon is thus required.
Carbon dioxide: from being a problem to being the solution
If CO2 becomes the industry’s carbon source, the carbon cycle will be closed. This means that additional emissions of CO2 will not take place. Keeping this in mind, this article presents a novel process based on membrane technology that captures CO2 from flue gases to produce pure sodium bicarbonate crystals (NaHCO3) [2].
Sodium bicarbonate is a white powdered salt with huge demand, such as baking powder and other food additives, used in soaps, detergents, pharmaceuticals, cosmetics, fire extinguishers, animal feed additives, and in the production of many other chemicals. Its market is projected to grow at a compound annual growth rate (CAGR) of 5.5% to reach US$2.053 billion in 2026 [3]. The development of greener alternatives to produce NaHCO3 is thus a smart strategy.
The novel process presented in this work consists of two main steps (Figure 1), i.e., the CO2 absorption step and the NaHCO3 purification step.
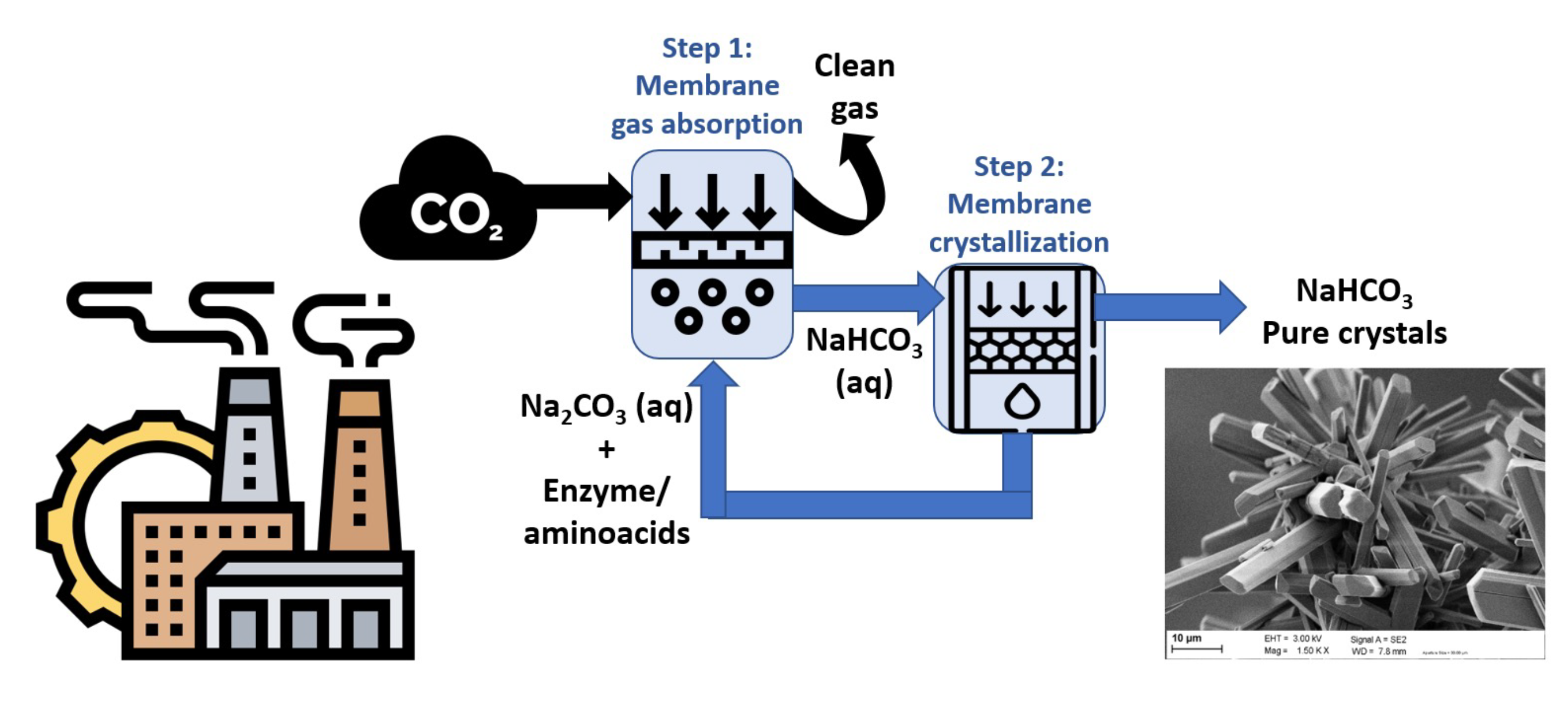
CO2 absorption
In the first step of the process, a gas stream proceeding from flue gases (environ 15 vol%CO2 in air containing impurities such as NOx and SO2) is sent to a membrane contactor in which the CO2 will contact a liquid solution containing sodium carbonate (Na2CO3) through the membrane pores. This non-dispersive gas-liquid contact allows a fast mass transfer with an easy operation in which the conditions of the gas and the liquid phases can be tuned independently. To enhance the mass transfer within the contactor, aminoacids and/or enzymes are used [4, 5]. The gas stream is thus cleaned (CO2 has been removed), and the liquid stream is rich in NaHCO3.
NaHCO3 purification
The bicarbonate solution is now passed to the second step so that pure sodium bicarbonate crystals of NaHCO3 are obtained. This crystallization step is performed in a membrane crystallizer. Several configurations of membrane crystallization can be used, depending on the type of energy available in the site [6]. A direct contact membrane crystallization process would be advised for those cases in which residual heat (temperature around 40-70°C) is available. In this case, the bicarbonate solution could be heated up so that water is evaporated within the membrane pores thanks to the thermal driving force. A clear advantage of direct contact membrane crystallization is the low energy consumption and pure water production as permeate. Once saturation concentration of the bicarbonate solution is achieved, crystal nucleation will happen within the membrane contactor to continue afterwards in a crystallization reservoir for further growth of sodium bicarbonate crystals.
Another option to perform the crystallization of NaHCO3 is via vacuum membrane crystallization, in which a vacuum is applied in the permeate side to create a huge driving force that will evaporate the water. Here pure water is also produced, and the evaporation rate is remarkably high.
Finally, the third kind of strategy to achieve the crystallization of NaHCO3 is by using osmotic membrane crystallization. If a high-concentrated solution of salts (osmotic solution) is available on site, for example, desalination brines, if one thinks of a potential process that integrates water desalination and CO2 capture, it would be possible to recover the energy intrinsically existing in the desalination brine to evaporate the water thanks to the osmotic driving force. The membrane separates the brines from the crystallizing solution, and water is evaporated until supersaturation is achieved.
These three membrane crystallization processes will lead to pure NaHCO3 crystals with high industrial interest.
Integrating CO2 capture and crystallization process in the industrial context to reduce global emissions
The described process is an alternative to the conventional NaHCO3 production
routes (i.e., the Solvay process and soda ash carbonation from trona), which are very intensive energetically and limited geographically in the case of using trona. This novel process provides a continuous system to produce NaHCO3 directly from Na2CO3 and CO2 from flue gases. CO2 is the source of carbon.
Footnotes
- Materials & Process Engineering (iMMC-IMAP), UCLouvain, Place Sainte Barbe 2, B-1348 Louvain-la- Neuve, Belgium
- Research & Innovation Center for Process Engineering (ReCIPE), Place Sainte Barbe 2, bte L5.02.02, B-1348 Louvain-la-Neuve, Belgium
References
[1] 2030 Climate Target Plan, European Commission, 2020.
[2] P. Luis, Continuous process and system for the production of sodium bicarbonate crystals. PCT/EP2021/084134; WO 2022/117800.
[3] Sodium Bicarbonate Market – Growth, Trends And Forecast (2020 – 2027) By Types, By Application, By Regions And By Key Players: Solvay, Church & Dwight, Natural Soda, Novacarb, https://www.industrydataanalytics.com/reports/global-sodium-bicarbonate-market.
[4] Molina-Fernández, C., Luis, P. Immobilization of carbonic anhydrase for CO2 capture and its industrial implementation: A review, Journal of CO2 Utilization, 47, art. no. 101475, 2021.
[5] Sang Sefidi, V., Luis, P., Advanced Amino Acid-Based Technologies for CO2 Capture: A Review, Industrial & Engineering Chemistry Research – Vol. 58, no.44, p. 20181- 20194, 2019.
[6] Sparenberg, M-C., Hanot B., Molina-Fernández C., Luis P. Experimental mass transfer comparison between vacuum and direct contact membrane distillation for the concentration of carbonate solutions. Separation and Purification Technology 275, 119193, 2021.
The author acknowledges the European Research Council (ERC) for the funding received under the European Union’s Horizon 2020 Research and Innovation Programme (Grant Agreement ERC Starting Grant UE H2020 CO2LIFE 759630).

This work is licensed under Creative Commons Attribution-NonCommercial-NoDerivatives 4.0 International.