THz and millimetre wave frequency techniques, combined with thin film measurement methods, provide early detection of corneal edema associated with disease in the view of Zachary Taylor, Assistant Professor at Aalto University
The adult human body is ~60% water. Tissue water content (TWC) varies from from 50%- 80% depending on age, location on the body, physiologic state, and so on. Many diseases can perturb this fraction and medical research today has dedicated profound time and resources developing diagnostic systems, tools, and algorithms to detect and characterise these abnormal changes in TWC. A system familiar to many is magnetic resonance imaging (MRI), which uses precisely tuned magnetic fields and RF pulses to form volumetrically resolved maps of body water content. Brain tumour imaging, blood perfusion analysis, and spinal joint assessment are among the countless possibilities with MRI generated TWC maps. However, magnetic fields are difficult to characterize at boundaries between tissue and air, therefore, quantitative assessment of surface tissue water content is extremely difficult.
One important, water-rich, surface tissue system not currently accessible with MRI is the cornea. The cornea is the outer most layer of the human eye (Figure 1) and principally composed of collagen and water in mass fractions of ~ 21% and 79% respectively. The tough but optically transparent tissue protects the delicate structure of the eye from the outside environment and is responsible for the majority of the focusing power of the human optical system. The bottom layer of the cornea (endothelium) sits adjacent to a water volume called the aqueous humour (Figure 1). The role of the endothelium is to actively pump water from the cornea and back into the aqueous humour to maintain 79% water and, therefore, ensure optical clarity. Perturbations from the 79% water lead to significant vision degradation.
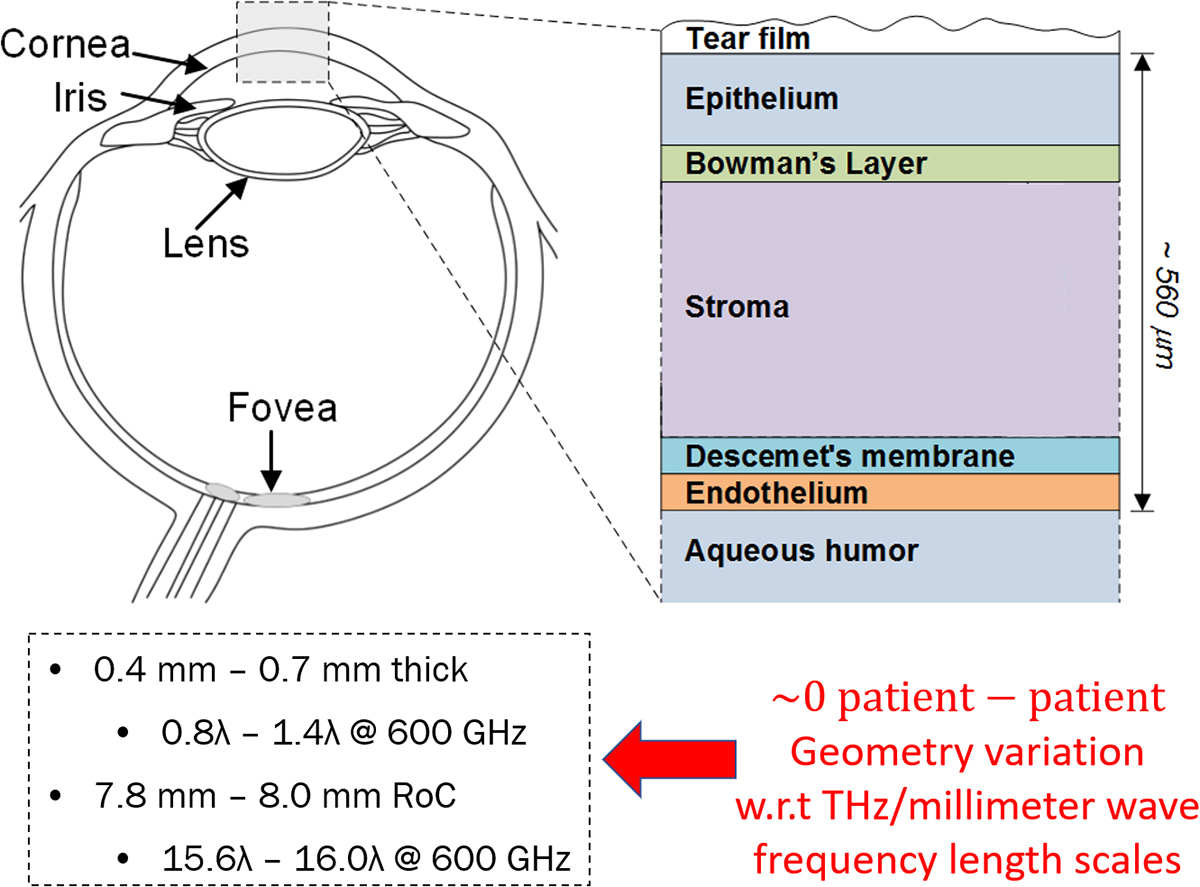
A common disease that attacks the endothelium’s pumping efficiency is Fuchs endothelial corneal dystrophy which affects ~40% of the world’s population over 40 years of age. A reduction in pumping efficiency leads corneal hyperhydration and then corneal edema due to uncontrolled diffusion of water from the aqueous humor to the stroma (Figure 1). Edema is readily apparent from increased corneal thickness and significant optical opacity. This gives the cornea a “milky” or glazed over appearance. Corneal edema and, therefore, disease detection via visual assessment of opacity is straightforward but it is difficult to treat the disease at this point in the progression.
The definitive treatment for Fuchs dystrophy is called lamellar transplant, where part or all of the diseased cornea is resected and donor or artificial cornea are grafted on. Graft rejection following transplant is not uncommon (1%-12% depending on surgical technique) and failure is typically preceded by the presence of edema in the graft. Detection of graft rejection via visual assessment of corneal edema is too late in the rejection progression to save the graft. Early and accurate detection and quantification of corneal edema, before perceptible changes in optical clarity, are not currently feasible. Development of sensitive and specific corneal edema measurement systems will considerably improve patient outcomes.
Despite efforts in research the clinical gold standard for water content is still pachymetry or a centre of the cornea thickness (CCT) measurement. This method recognises that an edematous cornea must expand (thicken) to make room for excess water and thus a history of steadily thickening cornea indicates compromised corneal health. However, the vast majority of patients do not have detailed CCT histories.
Further, sincepachymetry is an indirect measure of water it cannot, in a single time point, ascertain or even infer water content. Studies performed on patients with corneal disease suggest that small, initial increases in CTWC do not produce statistically significant increases in CCT. Some high-resolution microscopy studies have identified changes in cellular morphology in Descemet’s membrane (Figure 1) but these are exploratory and the clinical utility is unclear. What is needed is a direct measurement of CTWC that can be decoupled from variations in tissue geometry/morphology. THz and millimetre wave techniques may provide the key.
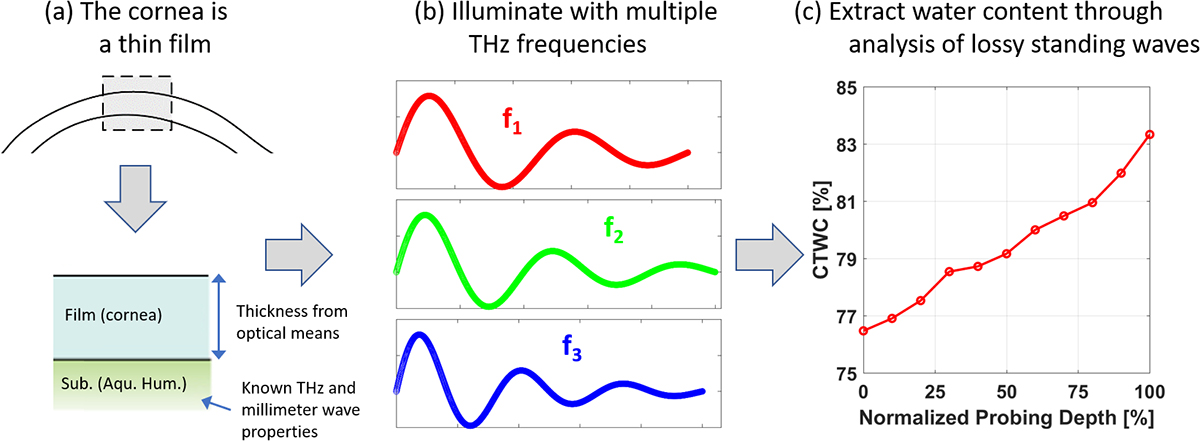
The inspiration for THz and millimetre wave probing of CTWC r content arose from ellipsometry; a measurement technique used to characterise thin films of materials. Ellipsometry illuminates the target with a laser that (1) has a wavelength on the order of the film thickness, and (2) the speed of light of that wavelength in the film is a very strong function of the film material and any imperfections in the film material. The illumination will bounce around inside the film and create a standing wave. Small changes in illumination parameters, such as wavelength, or angle of incidence create large, detectable changes in the standing wave. It is straightforward to numerically extract the thickness of the film and/or the material properties of the film simultaneously with a very high degree of accuracy.
The cornea is a perfect representation of a thin film (Figure 2) at THz and miliimeter wave frequency length scales. It sits on top of a known material with known properties (water) and its physical thickness can be accurately measured via a number of optical techniques. Three key features render cornea a great match to THz and millimetre wave imaging. First, the thickness range, 0.4 mm-0.7 mm (Figure 1), falls right in the middle of our free space THz wavelength range: 0.1 mm-3 mm. Second, the standard deviation in radius of curvature (RoC) is fractions of a wavelength in the band of interest which enable THz and millimeter wave systems to always assume the cornea is a sphere thus allowing for straightforward optical design.
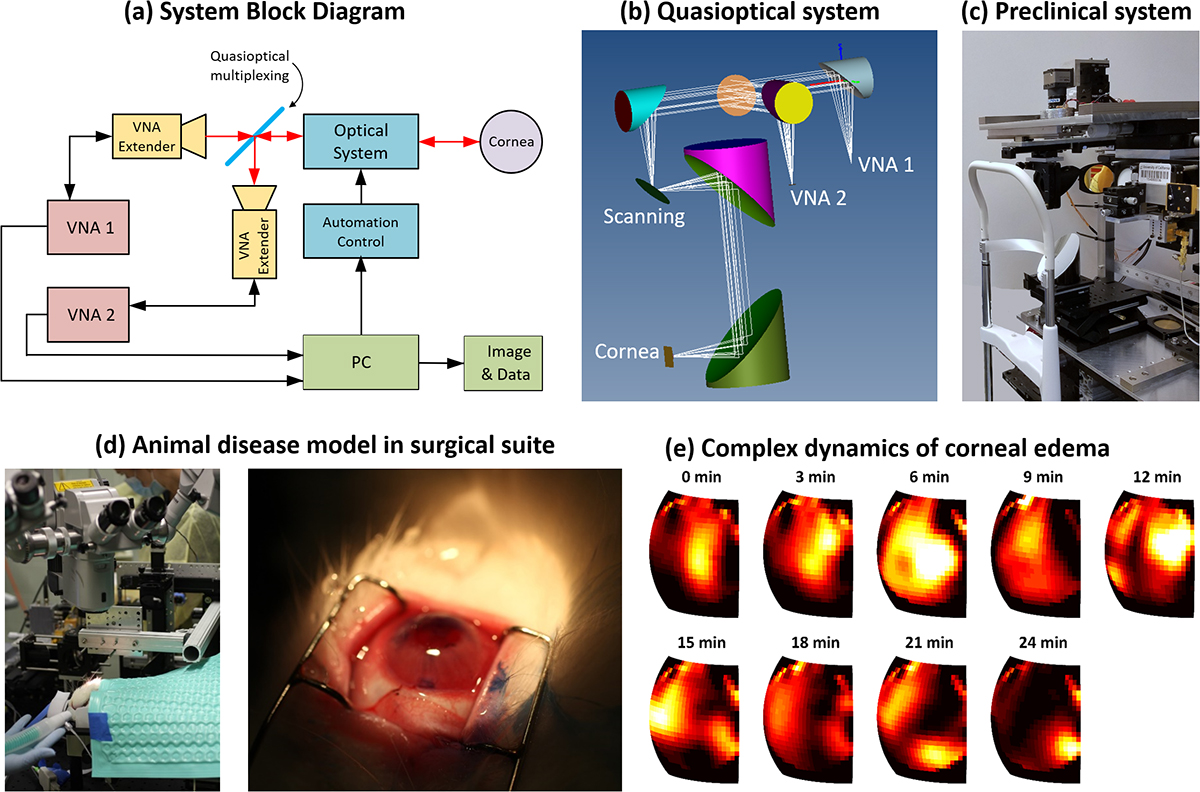
Finally, the propagation speed of THz and millimetre wave radiation through physiologic material is an extremely strong function of water content and a weak function of the non-aqueous components (e.g. collagen). Therefore, quantitative mapping of corneal water content , and even water content gradients, can be through resolving the standing waves created by illuminating with an ensemble of frequencies at in the THz and millimetre wave bands (Figure 2).
Our programme was established by an R01 grant (R01EY021590) from the National Eye Institute (NEI) for endothelial disease and graft rejection detection. We were the first to introduce the concept of lossy etalon analysis to THz millimetre wave imaging of cornea and the first to use it to decouple CCT changes from CTWC perturbations in vivo. We are currently able to resolve ~1% water by volume changes in corneal phantoms and are close to demonstrating this capability in in vivo animal models.
A current snapshot overview of the programme is shown in Figure 3. We are building multi-transceiver systems at complementary frequency bands to enhance water gradient extraction (Figure 3(a)) and have performed extensive optical simulations to optimise signal acquisition from curved surfaces (Figure 3(b)). Numerous human imaging systems prototypes have been built (Figure 3(c)) and are currently being tested tested on volunteers. We have also completed surgical trials in rabbit models (Figure 3(d)) and observed corneal edema formation (Figure 3(e)) after removal of Descemet’s membrane (Figure 1).
Corneal tissue water content (CTWC) quantification offers a unique opportunity for THz and millimetre wave techniques in medicine. The relative lack or physiologic variation in corneal morphology on THz length scales and the extremely strong dependence of THz properties on water content enable successful application of thin film metrology. Successful clinical translation of this technology will require a multidisciplinary approach combining the fields of RF remote sensing, antenna design, radar engineering, optical techniques. In recognition of these challenges and the broad range of expertise required, we has moved the entire corneal tissue water content imaging programme to Aalto University in Finland.
Please note: This is a commercial profile
Zachary Taylor
Assistant Professor
Aalto University
Tel: +358 050 595 5617
www.aalto.fi/department-of-electronics-and-nanoengineering/millimetre-wave-and-thz-techniques
Editor's Recommended Articles
-
Must Read >> Innovative device for cataract surgery in sight
-
Must Read >> How is blockchain disrupting health and wellness?