Prof Chris Barnes & Dr Jack Rutter share a future vision for microbiome engineering – harnessing the microbiome’s role in health & disease. Translating the microbiome requires investment in new physical, statistical, artificial intelligence & engineering biology principles, they argue
The human body contains vastly complex ecosystems of microbes inhabiting almost every available niche. The microbiome has been implicated in numerous human health problems, including diabetes, obesity and inflammatory bowel disease; associated with several mental health and neurological issues such as depression, anxiety, autism, Parkinson’s and Alzheimer’s; the metabolic modification of drugs increasing toxicity or impairing efficacy; and is now known to modulate patient response to — and recovery from —surgery. Understanding the host-microbiota complex system, how it affects health and how we can manipulate it, is a great challenge.
Microbiome engineering will leverage fundamental engineering principles to modulate host-microbiome systems for therapeutic interventions. The development of physical theories and an engineering toolbox for microbiome manipulation promises exciting therapeutic possibilities, combining opportunities to improve existing therapies and develop completely new ones.
Imagine: microbial signatures that can provide early detection of heart disease and cancer; stratification of patients into groups that will respond to different therapies; live biotherapeutics that improve the efficacy and reduce the toxicity of existing drugs by co-modification of the microbiome; live biotherapeutics that can reverse the dysbiotic states associated with the chronic inflammation of metabolic diseases such as Type II diabetes and obesity; antibacterial agents for the treatment and alleviation of antimicrobial resistance; microbial gene drives to remove anti-microbial resistance in patients; smart autonomous cancer therapeutics from engineered bacteria that can co-locate with tumours and deliver chemotherapeutics. These are just some examples; the potential is unprecedented, with significant benefits for the ageing population, a problem of huge global importance.
We propose the development of an Institute of Microbiome Engineering (IME) with eight foundational themes (Figure 1). When combined with larger data sets and community standards for experimental studies, these themes will address the physical and engineering challenges underlying the engineering of host-microbiota systems.
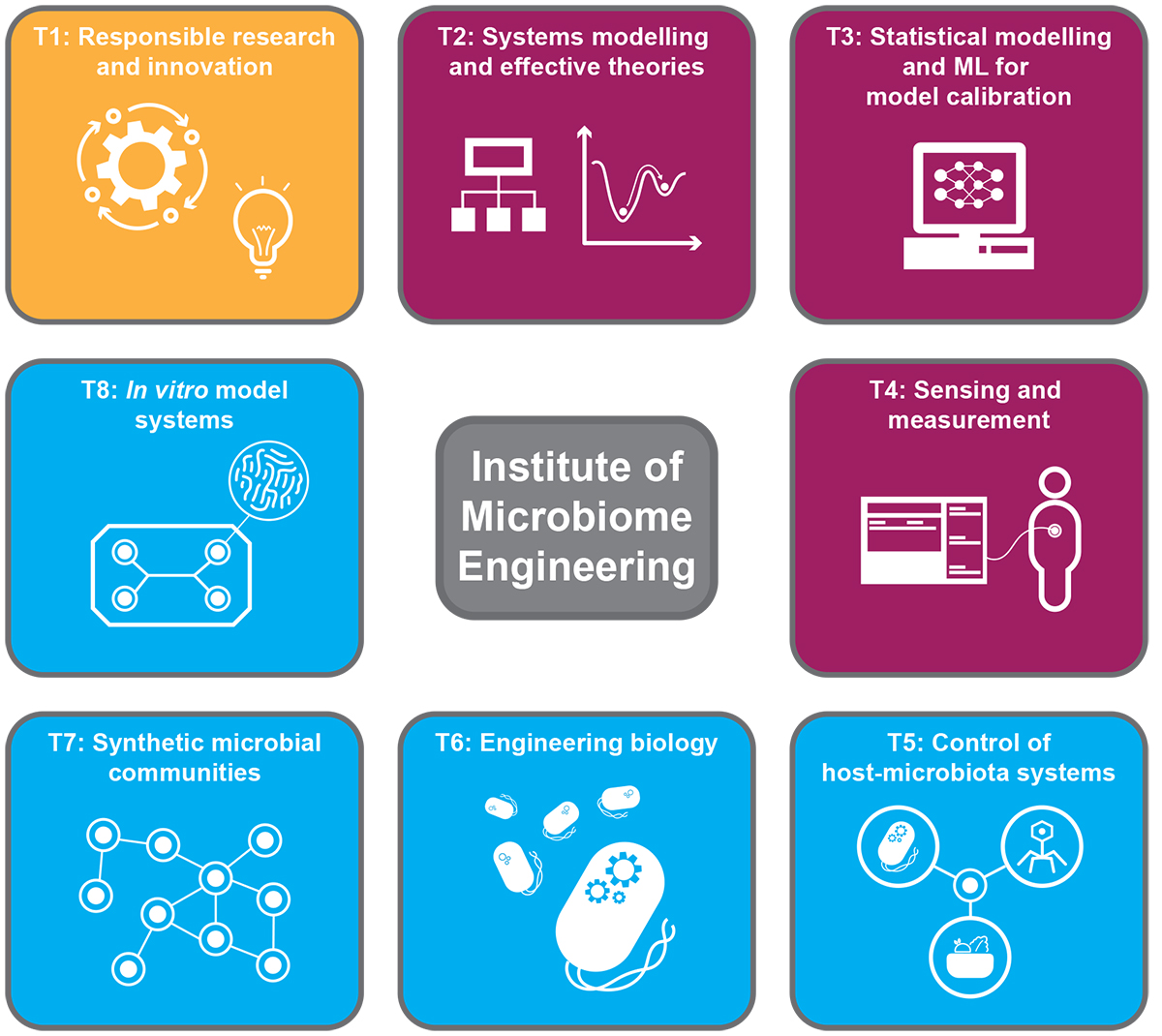
Theme 1: Responsible Research and Innovation (RRI):
RRI must be an integral driving force of microbiome engineering. We aim to create an ongoing societal dialogue, engaging stakeholders, including patients, clinicians, politicians, academics and the general public, addressing issues, including, ethics, equality, bias, risks and safety. This conversation will guide the direction of the institute.
Theme 2: Systems modelling and effective theories:
Mathematical models underlie all engineering disciplines and will allow for mechanistic/causal understanding and the prediction of therapeutic interventions. However, in standard approaches, the number of parameters grows super-linearly with the number of species. New mathematical and statistical physics approaches for the construction of approximate or effective theories are required to capture the essential behaviour in a tractable manner.
Theme 3: Artificial intelligence and statistical inference for predictive modelling:
Mathematical models must be fit to data. However, current data are limited due to being sampled at a low number of time points. Contrast this with the wealth of data measured for weather prediction. Current methods do not correctly account for these uncertainties and will give us false and misleading information about the underlying community structure. Bayesian and AI approaches to inference of microbial network models, which leverage existing data sets, are required.
Theme 4: Sensing and measurement:
New tools for interrogating the microbiota will be needed to inform mathematical models for understanding and prediction. These will include technologies based on mass spectrometry and imaging methods such as ultrasound. Another avenue is point-of-care (POC) devices that produce rapid and low-cost estimates of patient microbiota and metabolome. These low-cost devices could also be used at home to monitor different microbiota conditions and potentially save the global healthcare industry billions of dollars in unnecessary diagnostic treatments.
Theme 5: Control of complex host-microbiota system:
Microbiome engineering aims to control microbiota species abundance in addition to modulating emergent properties such as transitioning from dysbiotic to homeostatic states. This will use precise nutritional factors or live therapeutics, including phages, probiotic formulations and engineered microbes. These approaches will require new methods based on control theory and AI to understand optimal control of the microbiota.
Theme 6: Engineering biology: Robust designs and feedback control:
Synthetic biology will develop new phage and bacterial therapeutics incorporating effector agents that can
manipulate and perturb microbiota interactions. The implementation of engineered biological systems in an environment such as the gastrointestinal tract makes the requirement for robust design processes and self-regulatory feedback mechanisms critical to ensure predictable performance. This requires new engineering approaches to incorporate robustness into the design-build-test-learn cycle.
Theme 7: Synthetic microbial communities:
The emergent properties and information processing capacities of open microbial ecological systems remain unexplored. By building synthetic community models, we can study adaptability, structural stability, dynamics, self-organisation and tipping points.
Theme 8: In vitro model systems:
The use of microscale engineering technologies can be combined with cultured living human cells and microbiota to create model systems that recapitulate the physiological and mechanical microenvironment of the living gastrointestinal tract. These systems enable the study of complex human physiology and pathology in an organ-specific context, and they offer the potential to develop specialised in vitro human disease models that could revolutionise drug discovery and development.
Summary
The themes described here represent a future vision for harnessing the microbiota’s role in health and disease. Although there may be other challenges along the way, we argue that investment in foundational engineering principles is required to realise this endeavour. Without this solid foundation, research in this area will be hampered. Ultimately these tools will allow us to understand and manipulate the human microbiota, improving health outcomes that underpin healthy ageing.
Authors
Prof Chris Barnes is a Professor in the Division of Biosciences within the Faculty of Life Sciences at University College London and Head of the Computational Systems and Synthetic Biology (CSSB) group. His interdisciplinary career has spanned multiple fields, including particle physics, genetics, statistics, computational systems biology and synthetic biology.
Dr Jack Rutter is a Postdoctoral Research Associate in the CSSB group. His research looks at how we can use the techniques of synthetic biology to engineer the human microbiome for diagnostic and therapeutic purposes.
Please note: This is a commercial profile