This chemistry focus delves into making nanocellulose filters for water purification with underutilised biomass, as described here by Benjamin Hsiao from Stony Brook University in the U.S.
Clean water supply, a sustainable food source, power generation and storage for rural electrification, and information access/exchange are all recognised as critical components for commercial, educational and social development in off-grid communities around the world. Among these components, access to clean drinking water is a particularly pressing issue for 1.8 billion people worldwide and over 1.5 billion are afflicted with water-related diseases annually. (1) This issue is continually growing worse, with global problems like rapid population growth, climate change, natural disasters and pollution all compounding the threat that many more people will face water insecurities. Existing solutions require expensive energy sources and highly manufactured synthetic materials, which make the technologies inaccessible and unsustainable to those needing them the most, especially those in off-grid communities.
Solving the drinking water challenge
One possible solution to tackle the drinking water challenge is based on the convergence of chemistry, botany, engineering, economics, sociology, policy and education. For example, by using underutilised local biomasses, from agriculture waste to invasive species, we can create renewable, low-cost and efficient purifying materials to deal with local drinking water problems or treat agriculture/aquaculture wastewater, and those same local biomasses can be further processed into durable and environmentally friendly consumer products or fertilisers for crop growth to provide new economic opportunities within the communities. Although the usefulness of nanotechnology for water remediation has been known for over a decade, only within the last few years have researchers realised the potential of using nanoscale cellulose (nanocellulose) for varying water purification applications (2), including membrane filtration.
Cellulose is the most abundant natural polymer on earth. It is produced by higher plants, a wide range of varying bacteria, algae and fungi, and certain animals such as tunicates. (3) Both wooden and non-wooden plant fibres can be used to extract nanocellulose. A general consensus is that non-wooden plants usually have a lower lignin content than wooden plants, which make these non-wooden plant fibres easier to delignify, thus facilitating the nanocellulose extraction process.
Nanocellulose
Current chemistries for nanocellulose extraction have mainly been developed to deal with wooden plants, and for lower value non-wooden plants the major focus today is the production of biofuels. (4) As the logistics of collection, transportation and decortication for biofuel production are being worked out to deal with agricultural residues (which are utilised mainly as a burning source for waste removal or energy generation and cause undesired air pollution) (5), the exploration of new and simple chemistries that can extract nanocellulose from non-wooden plants in a more cost-effective and environmentally friendly manner will be very valuable. These new chemistries will definitely enable us to develop low-cost nanocellulose processes to upcycle vastly underutilised non-wooden biomass, especially for water purification.
In this article, we provide the perspectives of using nanocellulose as a valuable building block in the fabrication of low-cost and highly efficient water filtration membranes. Membrane technology remains to be the most energy-efficient process to remove contaminants (from micron size particles to angstrom size hydrated ions) from water.
However, the current membrane technology, involving relatively expensive synthetic materials, is often non-sustainable for the poorest communities in our society. It is conceivable that nanocellulose membranes developed from inexpensive, abundant and sustainable resources (e.g., agriculture residues and underutilised biomass waste) can lower the cost of membrane separation as these membranes offer the ability to remove a range of pollutants in one step, via size exclusion and/or adsorption. The nanocellulose enabled membrane technology is not only suitable to tackle the global drinking water challenges, but can also provide a new low-cost platform for varying pressure-driven filtrations, such as microfiltration (MF) and ultrafiltration (UF). We believe nanocellulose enabled membrane technologies are only in the initial stage of development and they will grow rapidly in the future.
We also note that these technologies can take advantage of the existing paper/packaging processes, although the final products (stable membranes with controllable pore size and porosity) for water filtration will have very different requirements and considerations.
One example of a gravity-driven MF system that can simultaneously remove most common harmful bacteria (e.g. Escherichia Coli, Hepatitis A, Salmonella, Cryptosporidium) as well as smaller-scale viruses from water is illustrated in Figure 1.
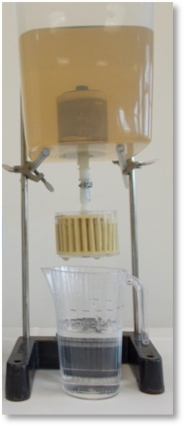
As the filtration operation is driven by gravity, the system is low-cost, easy to maintain and suitable for the off-grid environment. The membrane design for this filtration system is as follows. Microscale cellulose fibres are first chemically crosslinked to form a water stable scaffold, where the void in the microfibrous scaffold is then filled with nanocellulose (Figure 2). The infusion of different amounts of nanocellulose into the scaffold is an effective way to finetune the average pore size of the membrane without drastically decreasing the porosity. For the gravity-driven MF membranes, the average pore size is usually controlled at around 0.2 μm, which is sufficient to stop the passage of bacteria, whereas the presence of nanocellulose with the electrostatically charged surface can further offer the adsorption capability to remove viruses and toxic metal ions
In Figure 2, we depict another membrane format, where a three-layered thin-film nanofibrous composite (TFNC) structure, containing cellulose fibres of different diameters is shown. The pore size of the barrier layer in the TFNC membrane is directly related to the diameter and the layer thickness of nanocellulose used. This membrane format is useful for ultrafiltration where the average pore size can be reduced down to a few nanometres, in the same range of nanocellulose diameters. Due to the nature of the non-woven structure of the composite membranes, and the hydrophilic nature of cellulose, such a membrane system usually offers high permeation flux and lower fouling tendency in UF operations.
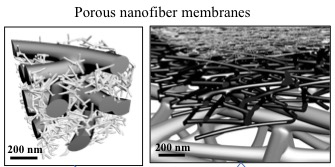
Currently, large-scale commercial nanocellulose production technologies are primarily based on wood-based biomass. Although they have many advantages in terms of logistics and capacity for mass production, the use of cheaper processes and underutilised non-wood biomass, such as agricultural residues, for smaller-scale production with properties (e.g. dry and wet membrane strength, membrane pore size and porosity, long-term stability in aqueous medium) tailored for water purification, will be particularly useful for developing countries.
References
- WHO/UNICEF, 2015.
- A. W. Carpenter, C. F. de Lannoy, M. R. Wiesner, Environ. Sci. Technol. 2015, 49, 5277.
- R. H. Atalla, in Comprehensive Natural Products Chemistry,(Ed: B. M. Pinto), Elsevier, New York, USA 1999.
- M. Ali, M. Saleem, Z. Khan, I. A. Watson, in Biomass, Biopolymer-Based Materials, and Bioenergy, (Eds: D. Verma, E. Fortunati,
- Jain, X. Zhang), Woodhead Publishing, 2019, 369.
- T. Ahmed, B. Ahmad, The Pakistan Development Review 2014, 53, 275.
Please note: This is a commercial profile