Dr Amparo Güemes, an award-recognised woman in science and engineering, describes her research for an efficient closed-loop system for diabetes management
Diabetes is a widespread chronic metabolic disease affecting a substantial number of individuals worldwide. In 2021, there were approximately 537 million people living with any type of diabetes globally, out of which around 8.7 million people live with type 1 diabetes (1).
By 2030, it is projected to become the 7th leading cause of death (2). In the United Kingdom, the prevalence of diabetes is significant, with around 4 million people currently affected and an estimated 10% of which have Type 1 diabetes (T1DM) (3).
A recent report by Diabetes.co.uk has highlighted the significant financial burden on the NHS imposed by diabetes, accounting for over £1.5 million per hour or approximately 10% of the NHS budget for England and Wales (4,5).
In total, the annual cost of treating diabetes and its complications amounts to a global estimate of US $966 billion in 2021 for adults aged 20-79 years and £14 billion solely in the UK (1). Notably, a significant portion of this expenditure is attributed to the treatment of complications, which incurs considerably higher costs.
Exploring state-of-the-art technology for diabetes management
Within the realm of diabetes research, my research delves into the understanding of a widely recognized disease characterized by disrupted glucose levels from a different perspective: neuromodulation.
Diabetes is a chronic metabolic condition wherein elevated blood glucose levels occur due to dysfunctional insulin secretion or impaired insulin action. In a healthy state, the pancreas’ beta cells release insulin, enabling the body to store and utilize sugar derived from carbohydrates.
However, in diabetes, these processes are disrupted, either through autoimmune destruction of beta cells leading to type 1 diabetes or insulin resistance in tissues resulting in type 2 diabetes.
The current state-of-the-art technology for diabetes management is the artificial pancreas, a closed-loop insulin delivery system that integrates a continuous glucose monitor (CGM), an insulin pump, and a sophisticated control algorithm.
Advancements in technology have led to the evolution of minimally invasive and portable systems
This algorithm calculates the optimal insulin dose to inject based on glucose readings obtained from the sensor. Its development traces back to the 1960s when the first closed-loop system, known as the Biostator, was introduced for the treatment of individuals with T1DM. The initial commercial device demonstrated the potential for tight blood glucose control.
However, its cumbersome size, invasive nature, and limited usability outside clinical settings posed significant challenges. Over the years, advancements in technology have led to the evolution of modern systems that are minimally invasive and portable, revolutionizing T1DM care.
Improved glycemic control of the artificial pancreas
Latest clinical trials have shown improved glycemic control of the artificial pancreas compared to traditional methods, such as finger pricks and manual insulin therapy using insulin pens (6). However, the development journey of the artificial pancreas (AP) technology has highlighted several critical challenges that needed to be addressed (7).
To begin with, the artificial pancreas lacks an anticipatory control component, meaning it cannot proactively adjust insulin delivery based on predicted glucose fluctuations.
Additionally, user input is required, particularly during meal intake, leading to delays in insulin action of over 15 minutes. Moreover, reliance on self-reported carbohydrate content increases the risk of misestimation, affecting accurate insulin dosing.
It also presents challenges in effectively managing large disturbances caused by meals and exercise, and ensuring the adaptability of the system to the wide variability in patients’ physiological behaviour. There are other technical challenges to be addressed such as improving the accuracy and reliability of continuous glucose monitors, and ensuring the safety of insulin pumps by implementing fault detection mechanisms.
Overcoming these challenges remains paramount in the ongoing pursuit of developing a robust and efficient closed-loop system for diabetes management.
Developing a closed-loop system for diabetes management
One limitation of current closed-loop systems arises from disregarding the important contribution of the nervous system in healthy glucose regulation (8). During my BSc in Biomedical Engineering, I witnessed the emergence of bioelectronic medicine, which leverages electrical interfacing with peripheral nerves to develop innovative therapeutic approaches through neuromodulation.
That sparked my interest to explore bioelectronic medicine to revolutionize the traditional therapeutic approach to diabetes. Since then, my research vision has embraced integrating neurotechnology for metabolic control and ultimately into diabetes management and treatment to improve patient outcomes and mitigate associated complications.
This interdisciplinary approach aims to tap into the body’s natural closed-loop metabolic and neural mechanisms to achieve personalized, anticipatory, and automated glucose regulation.
The ultimate goal of this long-term research is the development of a closed-loop neuro-pharmacological system that combines neural signals, glucose measurements, and advanced algorithms to transform diabetes management (9).
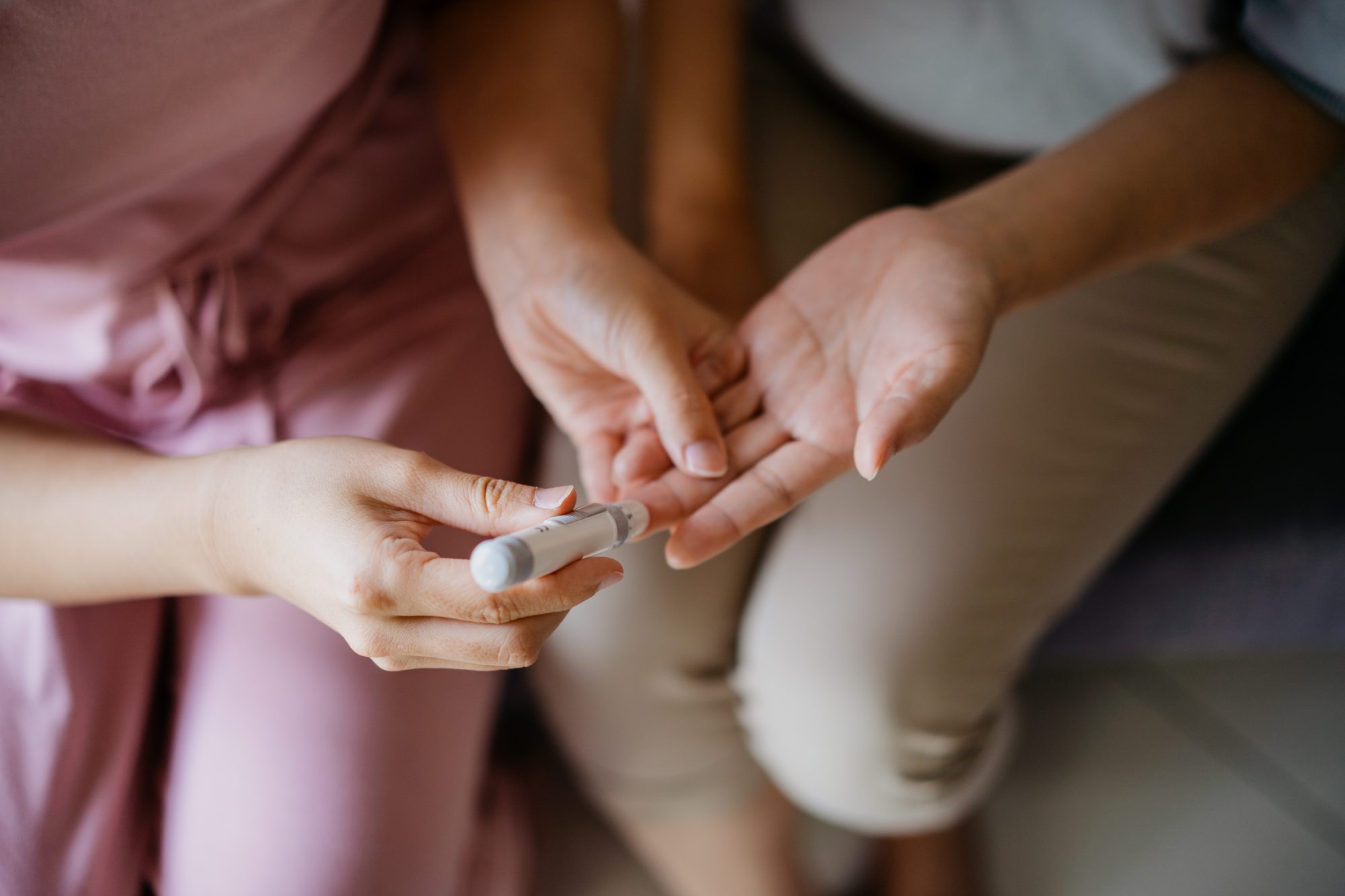
Neurotechnology to record and stimulate peripheral nerves
By utilizing neurotechnology to record and stimulate peripheral nerves, it becomes possible to obtain more accurate information about metabolic state and meal characteristics. This information can then be used to determine optimal insulin doses and deliver precise electrical stimulation to modulate glucose metabolism as needed.
Such a closed-loop platform has the potential to enhance patient autonomy, minimize delays in insulin action, and reduce the risk of misestimating carbohydrate content during meal intake.
Given the complexity and ambitious goals of our research, it is important to emphasize that this is a long-term project that requires a multidisciplinary step-by-step approach that integrates knowledge and techniques from different fields such as neurotechnology, computational modelling, bioelectronics and signal processing.
This approach provides versatility in problem-solving and has the potential for innovation and the discovery of new ideas.
To date, our focus has been on developing innovative technology and algorithms that facilitate a safe and effective interface with the nervous system to regulate glucose metabolism. The are four areas that serve as crucial starting points for this research journey.
The first critical component for the success of this project is the development of implantable electrical devices that can effectively interface with peripheral nerves and organs. This task poses significant challenges due to limited accessibility, invasiveness, and the risk of rejection.
However, the worldwide recognised Bioelectronics Lab led by Prof George Malliaras at the University of Cambridge has made substantial progress in designing and fabricating chronic devices that are flexible, conformable, and capable of matching the electrical and mechanical properties of the nerves (10).
Paving the way for precise and reliable neural interfacing
We further seek a seamless integration of the nervous system by incorporating PEDOT:PSS coatings, which decrease electrical impedance and improve the stability, biocompatibility and long-term performance of implantable bioelectronic devices. By addressing the challenges associated with implantable devices, we are paving the way for precise and reliable neural interfacing, which is essential for achieving accurate glucose control.
Another key area of our research focuses on neural recording and the analysis of neurograms, which are neural signals obtained from peripheral autonomic nerves. This area of study is currently scarce due to its complex nature.
Capturing, analyzing, and extracting information from neurograms
Neurograms present challenges such as their sub-millimetre size, low-amplitude waveforms, and non-stationary characteristics, which are often masked by noise. Our research aims to develop an optimal strategy for capturing, analyzing, and extracting information from neurograms under different metabolic states, with a specific emphasis on meal intake.
By improving our understanding of neural signals and their correlation with glucose levels, we can enhance the accuracy and reliability of closed-loop systems for glucose control.
Non-invasive or minimally invasive techniques for diabetes management
Furthermore, our research explores the modulation of glucose through nerve and/or organ stimulation. We have conducted preliminary studies using cervical vagus nerve stimulation (VNS) in healthy rodent models to investigate the impact of stimulation frequency on metabolic and hemodynamic responses.
However, this stimulation site has proven to be non-specific unless complex stimulation paradigms and electrode placements are used, which have to be individually tuned. Stimulation of the wrong fibres in the cervical vagus nerve may result in risky confounding systemic effects.
Therefore, we are currently exploring non-invasive or minimally invasive techniques that hold promise for overcoming the limitations associated with invasive electrical stimulation. By investigating targeted and precise stimulation approaches, we aim to refine our understanding of glucose metabolism modulation and pave the way for more effective and targeted interventions.
Finally, by combining computational modelling with experimental approaches, we can accelerate the progress of bioelectronic medicine. Computational modelling enables us to investigate the behaviour of bioelectronic devices in different scenarios and predict their performance before experimental implementation.
Bioelectronic interventions and glucose regulation
It allows for virtual testing of various parameters, such as electrode configurations or stimulation patterns, saving time and resources in the experimental phase. These models therefore facilitate the optimization of device parameters to ensure safe and effective operation, taking into account factors like power consumption, signal quality, and biocompatibility.
By simulating these intricate processes, we can better understand how bioelectronic interventions can influence glucose regulation and identify optimal strategies for achieving improved glycemic control.
Overall, our interdisciplinary research efforts allow the development of a more comprehensive and holistic understanding of metabolism control and its complex underlying mechanisms, helping to identify gaps in current knowledge and develop more effective interventions, ultimately leading to improved patient outcomes.
By addressing challenges related to implantable devices, neural recording and analysis, and glucose modulation through stimulation, we are laying the foundation for a comprehensive and personalized approach to glucose control.
Possibilities for treating other metabolic disorders
These foundational advancements will fuel our long-term vision of developing a closed-loop neuro-pharmacological system that transforms diabetes management and offers new possibilities for treating other metabolic disorders.
The potential research impact of this work is substantial and holds great promise for individuals with diabetes. Through the real-time analysis of metabolic and neurophysiological biomarkers, the proposed closed-loop technology aims to provide more precise and personalized diabetes management by replicating the body’s natural glucose regulation processes.
This has the potential to provide a more automated and anticipatory approach to glucose regulation, enhancing their quality of life and reducing the burden of daily self-management.
Beyond diabetes, the research conducted in this field can spark transformative advancements in healthcare. The integration of biological signal monitoring, bioelectronic medicine, and closed-loop systems opens doors to novel therapeutic approaches for other metabolic disorders and medical conditions.
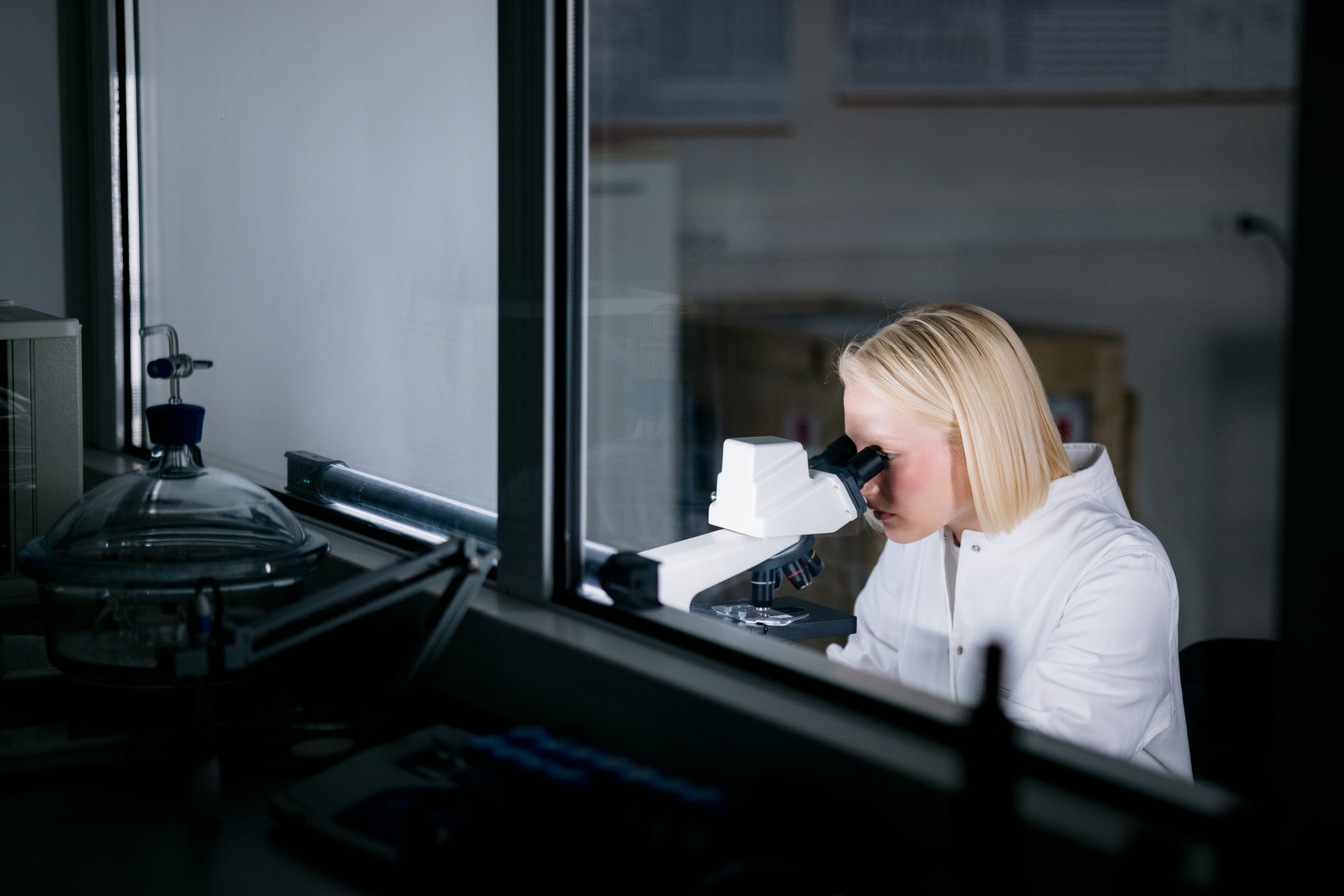
The insights gained from studying neural signals and their correlation with physiological processes can lead to breakthroughs in understanding and managing a wide range of diseases. This interdisciplinary research has the potential to shape the future of healthcare by enabling personalized, precise, and automated treatment strategies.
Designed hardware and algorithms with advanced control models
Looking ahead, future work will focus on integrating the designed hardware and algorithms with advanced control models that improve current glucose controllers, which will be then pre-clinically validated in diabetic animal models.
In the longer term, we will focus on refining and optimizing the closed-loop neuro-pharmacological system, conducting rigorous clinical trials to validate its efficacy and safety, and exploring its potential application in other metabolic disorders.
I am grateful for the support provided by the Royal Commission for the Exhibition of 1851 during the three-year postdoc I am currently undertaking at the University of Cambridge, which has played a crucial role in the advancement of my research.
The Commission’s funding, resources and continuous support have enabled me to pursue this ambitious project, conduct rigorous and high-quality in silico and pre-clinical studies, and make significant progress in developing new bioelectronic devices to understand the neuro-metabolic system.
Overall, this Research Fellowship has allowed me to progress as an independent early researcher by acquiring new experimental and theoretical knowledge and improving my professional skills to successfully pursue my goal of becoming a professor and leading a dynamic and diverse research laboratory.
The 2023 L’Oréal-UNESCO UK and Ireland For Women in Science Rising Talent Award in Engineering
Furthermore, I am honoured to have received the 2023 L’Oréal-UNESCO UK and Ireland For Women in Science Rising Talent Award in Engineering, and I feel deeply grateful for the recognition it brings to my work and to the contributions of women in science.
This recent acknowledgement is a significant milestone in my journey and has further fuelled my passion and dedication to pushing the boundaries of knowledge in diabetes management. The Award will provide me with opportunities for networking, collaboration, and visibility within the scientific community, strengthening my resolve to make a meaningful impact in the field.
‘This award is An inspiration for other women pursuing careers in science and STEM’
As a woman in engineering, I feel privileged to receive this award and believe it serves as an inspiration for other women pursuing careers in science and STEM.
The prevalence and impact of diabetes on individuals and healthcare systems worldwide provide a driving motivation for research in this field. This project is an ongoing endeavour that requires continuous collaboration, interdisciplinary efforts, and the integration of cutting-edge technologies.
Through persistence, innovation, and a dedication to improving diabetes management, we are confident that our neuro-metabolic research will contribute to transforming the closed-loop treatment landscape and ultimately provide improved personalized treatment for people living with diabetes.
References
- International Diabetes Federation. IDF Diabetes Atlas, 10th edn. Brussels, Belgium: International Diabetes Federation, 2021.
- World Health Organization, Organization WH. (2016) Global report on diabetes 2016. 978: 88.
- https://beyondtype1.org/type-1-diabetes-statistics/ [Last accessed June 2023]
- https://www.diabetes.co.uk/cost-of-diabetes.html [Last accessed June 2023]
- Stedman M, Lunt M, Davies M, et al Cost of hospital treatment of type 1 diabetes (T1DM) and type 2 diabetes (T2DM) compared to the non-diabetes population: a detailed economic evaluation BMJ Open 2020;10:e033231. doi: 10.1136/bmjopen-2019-033231
- Brown SA, Kovatchev BP, Raghinaru D, et al. (2019) Six-Month Randomized, Multicenter Trial of Closed-Loop Control in Type 1 Diabetes. N Engl J Med; 381: 1707–17.
- Bertachi, A., Ramkissoon, C. M., Bondia, J., & Vehí, J. (2018). Automated blood glucose control in type 1 diabetes: A review of progress and challenges. Endocrinología, Diabetes y Nutrición (English ed.), 65(3), 172-181.
- Güemes, A., Georgiou, P. (2018). Review of the role of the nervous system in glucose homoeostasis and future perspectives towards the management of diabetes. Bioelectron Medicine4(1), 9.
- Güemes Gonzalez A, Etienne-Cummings R, Georgiou P. (2020) Closed-loop bioelectronic medicine for diabetes management. Bioelectron Med; 6: 11.
- Khodagholy D, Doublet T, Gurfinkel M, Quilichini P, Ismailova E, Leleux P, Herve T, Sanaur S, Bernard C, Malliaras GG. Highly conformable conducting polymer electrodes for in vivo recordings. Adv Mater. 2011 Sep 22;23(36):H268-72.
- Güemes, A., Herrero, P., Bondia, J. Georgiou, P. (2019). Modeling the effect of the cephalic phase of insulin secretion on glucose metabolism. Medical & Biological Engineering Computing 1–14.
Editor's Recommended Articles
-
Must Read >> A changing landscape for type 1 diabetes
-
Must Read >> Diabetes: A Global Health Challenge